A Japanese research team has solved a quark-gluon plasma mystery that has long-evaded scientists and will significantly enrich our understanding of the Universe.
Since its discovery, enigmatic quark-gluon plasma has stimulated debate among the scientific community due to a discrepancy between theoretical modelling and experimental data that is unable to explain particle yields.
In their study, ‘Nonequilibrium components in the region of very low transverse momentum in high-energy nuclear collisions,’ researchers have innovated a new ‘dynamical core-corona initialisation framework’ to explain these high-energy nuclear collisions.
What is quark-gluon plasma?
Fundamental scientific research uncovered the existence of quark-gluon plasma – a type of matter known to be present in the early Universe – only a microsecond after the Big Bang. The Big Bang theory suggests that quark-gluon plasma filled the Universe before the creation of other matter that we know today.
Quark-gluon plasma is the building block of all matter in existence and is essentially a soup of quarks and gluons that is cooled down over time to create hadrons, such as protons and neutrons. For years, scientists have endeavoured to create that soup to further our understanding of the Universe’s inception.
The disparity between experimental data and theoretical modelling
In recent years, scientists have attempted to recreate the extreme conditions that quark-gluon plasma existed in through relativistic heavy-ion collisions – using accelerator facilities such as the Large Hadron Collider (LHC) and the Relativistic Heavy Ion Collider. This experimental data has enabled researchers to form a more comprehensive understanding of quark-gluon plasma.
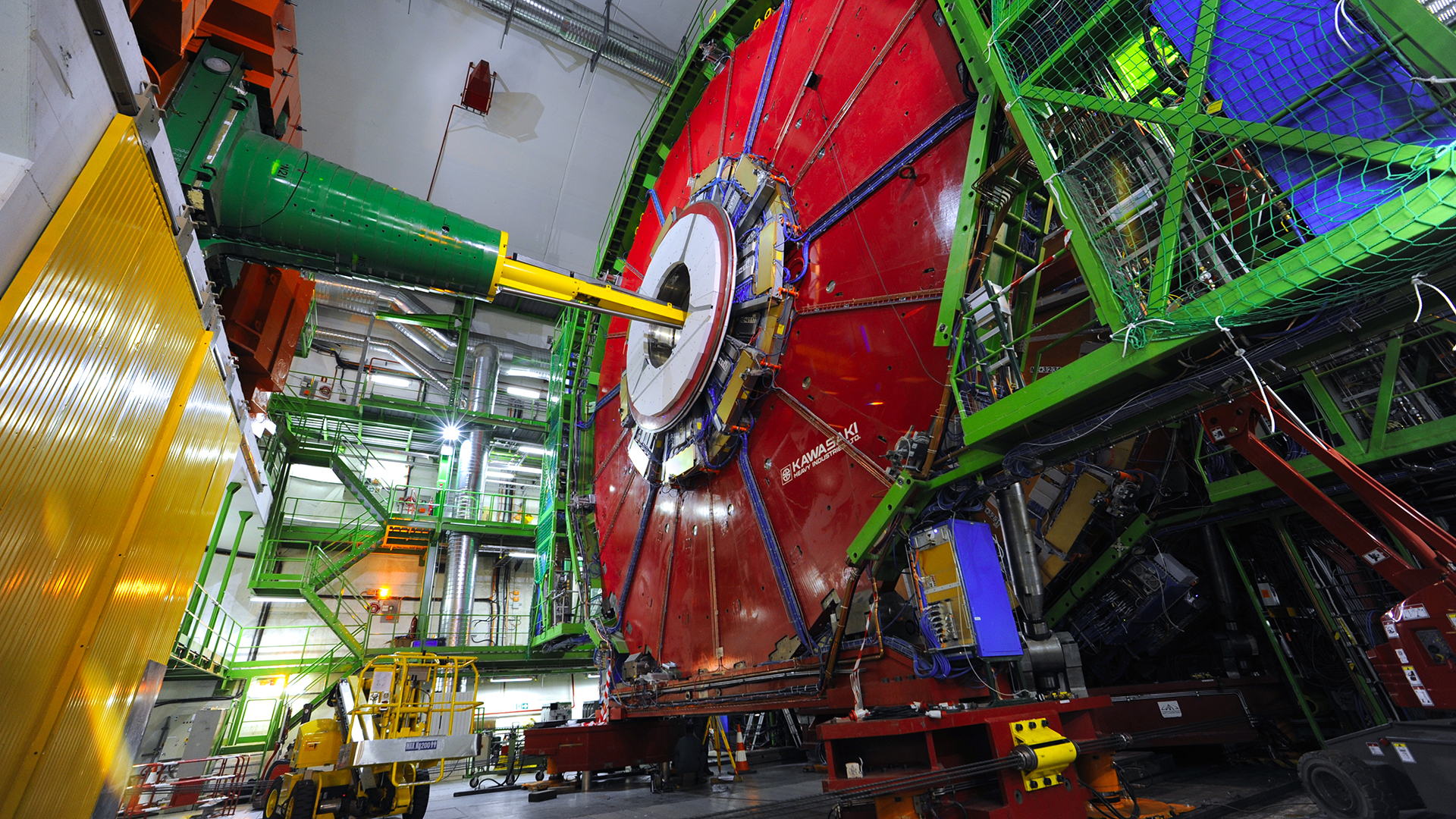
In contrast, due to the plasma behaving like a liquid, theoretical physicists have utilised multistage relativistic hydrodynamic models to explain the data. However, this has resulted in differences between these models and data with regard to low transverse momentum, in which the conventional and hybrid models fail to explain particle yields in the experiments.
The research team proposed an innovative method to remedy this deviation.
Professor Tetsufumi Hirano, the leader of the study and theoretical physicist at Sophia University, explained: “To find a mechanism that can account for the discrepancy between theoretical modelling and experimental data, we used a dynamical core-corona initialisation (DCCI2) framework in which the particles generated during high-energy nuclear collisions are described using two components: the core, or equilibrated matter, and the corona, or nonequilibrated matter. This picture allows us to examine the contributions of the core and corona components towards hadron production in the low transverse momentum region.”
Developing the dynamical core-corona initialisation framework
Using a simulation programme known as PYTHIA, the team performed heavy-ion Pb-Pb collisions at an energy of 2.76 TeV to evaluate the DCCI2 framework. The dynamical initialisation of the quark-gluon plasma fluids enabled the separation of core and corona components, which were caused to undergo hadronisation through switching hypersurface and string fragmentation, respectively. The hardons underwent resonance decays to obtain the transverse momentum (pT) spectra.
Dr Yuuka Kanakubo, a doctoral student at Sophia University, commented: “We switched off the hadronic scatterings and performed only resonance decays to see a breakdown of the total yield into core and corona components, as hadronic scatterings mix up the two components in the late stage of the reaction.”
The team analysed the fraction of core and corona components in the pT spectra of charged pions, charged kaons, and protons and antiprotons for collisions at 2.76 TeV. Subsequently, these spectra were compared to experimental data from the ALICE detector at LHC for Pb-Pb collisions at 2.76 TeV.
This allowed the researchers to calculate the contributions from corona components. Finally, they examined the effects of contributions from corona components on the flow variables.
What did the results reveal about quark-gluon plasma?
The researchers observed a relative increase in corona contributions in the spectral region of approximately 1 GeV for both 0-5% and 40-60% centrality classes. Although this was true for all hadrons, they discovered almost 50% corona contribution to particle production in the spectra of protons and antiprotons in the region of very low pT (≈ 0 GeV).
The results of complete DCCI2 simulations demonstrated better agreement with the ALICE experimental data compared to when only core components with hadronic scatterings were compared. Moreover, the corona contribution was discovered to be responsible for diluting the four-particle cumulants from core contributions, suggesting more permutations of particles with corona contribution.
Professor Hirano concluded: “These findings imply that the nonequilibrium corona components contribute to particle production in the region of very low transverse spectra. This explains the missing yields in hydrodynamic models, which extract only the equilibrated core components from experimental data. This clearly shows that it is necessary to extract the nonequilibrated components as well for a more precise understanding of the properties of QGP.”