A recent experiment at Fermilab has observed large asymmetry between the up and down sea quark distributions in the proton.
The first direct evidence for point-like constituents in the nucleons came from the observation of scaling phenomenon in Deep-Inelastic Scattering (DIS) experiments. These point-like charged constituents, called partons by Richard Feynman, were found to be spin-1/2 fermions. These partons were initially identified as the valence quarks in the Constituent Quark Models.
However, it was soon realised that valence quarks alone could not account for the swarm of low momentum partons observed in DIS. These low-momentum partons, dubbed wee-partons by Feynman, were interpreted as the quark and antiquark sea of the nucleon. DIS experiments, therefore, provided the first evidence for the existence of antiquarks in the nucleon.
The observation of partons in DIS experiments paved the road to the formulation of Quantum Chromodynamics (QCD) as the theory for strong interactions.
Nevertheless, the exact form of the parton distribution functions (PDF) cannot be deduced from perturbative QCD. Like many static properties of hadrons, the PDFs belong to the domain of non-perturbative QCD.
Despite the great progress in Lattice Gauge Theory (LGT) in treating the bound-state properties of hadrons, it remains a challenge to predict PDFs using LGT.
The early DIS data suggests that the nucleon consists of three quarks in a background of an infinite number of quark-antiquark pairs. The importance of the quark-antiquark pairs in the nucleon is in sharp contrast to the situation for the atomic system, where particle-antiparticle pairs play a relatively minor role.
In strong interactions, quark-antiquark pairs are readily produced as a result of the relatively large magnitude of the coupling constant αs, and they form an integral part of the nucleon’s structure.
There are at least two reasons why it is important to measure the parton distribution functions of the nucleons.
First, the description of hard processes in high energy interactions requires parton distribution functions as an essential input. Second, many aspects of the parton distributions can be compared with the predictions of theoretical models based on perturbative as well as non-perturbative QCD.
We present the status of our knowledge of the flavor asymmetry of the light-quark sea in the proton. The opportunities to further explore this subject at existing accelerators and the future Electron-Ion Collider (EIC) will also be discussed.
Flavor asymmetry from the DIS
The earliest parton models assumed that the proton sea was flavor symmetric, even though the valence quark distributions are clearly flavor asymmetric. Inherent in this assumption is that the content of the sea is independent of the valence quark’s composition. Therefore, the proton and neutron were expected to have identical sea-quark distributions. The assumption of flavor symmetry was not based on any known physics, and it remained to be tested by experiments.
Experiments with neutrino beams provided strong evidence that the strange-quark content of the nucleon is only about half of the up or down sea quarks. This flavor asymmetry was attributed to the much heavier mass for strange quark compared to the up and down quarks.
The mass for the up and down quarks being very similar suggests that the nucleon sea should be nearly up-down symmetric. A direct method to check this assumption is to compare the sea in the neutron to that in the proton measured in the DIS experiments.
Soon after the discovery of partons in electron-proton DIS, electron-deuterium scattering experiments were carried out to extract the electron-neutron DIS. The e−p and the e−n DIS data allowed a comparison of the up and down sea quark distributions in the proton. Despite the large uncertainty of the experimental data, Field and Feynman deduced from these data that the up and down sea quarks are different in the proton.
They further suggested that the Pauli blocking from the valence quarks would inhibit the down-quark sea more than the up-quark sea, hence creating an asymmetric proton sea.
However, the large experimental uncertainties prevented a definitive conclusion. A more precise DIS experiment was later carried out by the NMC Collaboration at CERN, showing that the up and down sea quark contents in the proton are different, at a confidence level of 4σ. The Drell-Yan process, described next, would provide a more direct and definitive measurement for this unexpected asymmetry of the proton sea.
Flavor asymmetry from the Drell-Yan Process
The first high-mass dilepton production experiment was carried out at the AGS in 1969, soon after DIS experiments were performed at SLAC. Drell and Yan interpreted the data within the parton model, in which a quark-antiquark pair annihilate into a virtual photon subsequently decaying into a lepton pair (see Fig. 1).
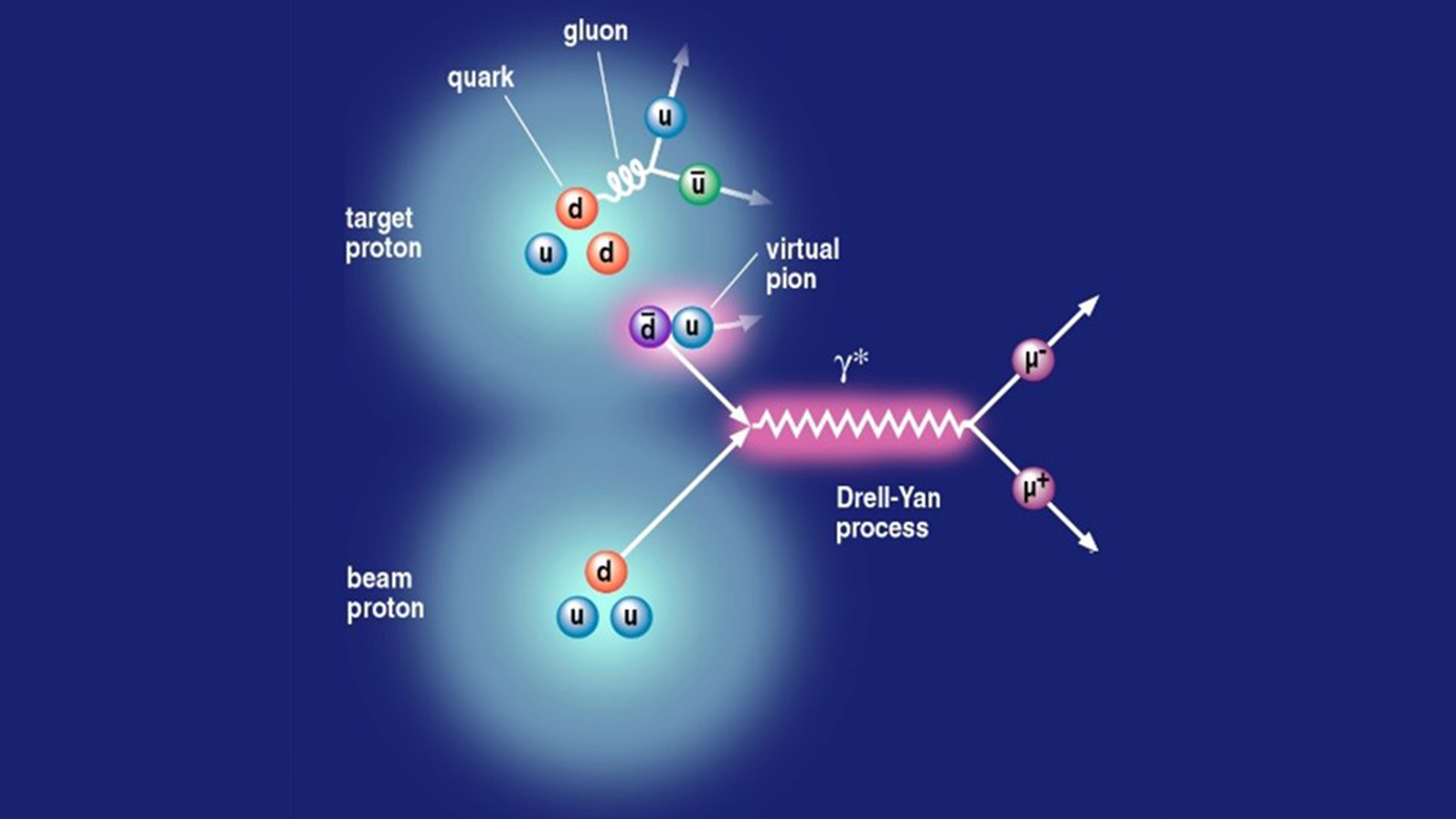
of the meson cloud in the target proton is highlighted.
This simple model could explain several pertinent features of the data, and the high-mass continuum lepton-pair production is, therefore, called the Drell-Yan (DY) process. Since the underlying mechanism for the DY process involves the annihilation of a quark with an antiquark, it is ideal for probing the antiquark contents of the beam or target hadrons.
Other than the DIS experiments, an independent and elegant method to test the flavor asymmetry of the light quark sea is the proton-induced Drell-Yan process. The Fermilab E772 Collaboration first compared the production of DY muon pairs from isoscalar targets to that from a neutron-rich target and set constraints on the nonequality of u ̅ and d ̅ in the proton.
Later, the CERN experiment NA51 carried out a comparison of the DY muon pair yield from hydrogen and deuterium and found that d ̅ is greater than u ̅ by a factor of two at x=0.18, where x is the fraction of the proton’s momentum carried by the antiquark.
At Fermilab, a DY experiment (E866/NuSea) aimed at a higher statistical accuracy with a much wider kinematic coverage than the NA51 experiment collected over 330,000 DY events, using three different spectrometer settings which covered the regions of low, intermediate and high mass muon pairs. The E866 experiment found that u ̅ is indeed different from d ̅ , as shown¹ in Fig. 2. By covering a broad kinematic range, the E866 experiment revealed an intriguing x dependence on the d ̅ (x)/ u ̅ (x) asymmetry. At the lowest x values, the u ̅ (x) and d̅ (x) are comparable. As x increases, d ̅ (x)/u̅ (x) rises monotonically, reaching a maximal value of about 1.75 at x ≈ 0.15. At even higher x values, the E866 data indicate that d ̅ (x)/u ̅ (x) starts to drop, becoming less than unity at the highest x value. The flavor asymmetry between u̅ (x) and d ̅ (x) was also observed by the HERMES collaboration using semi-inclusive deep inelastic scattering.
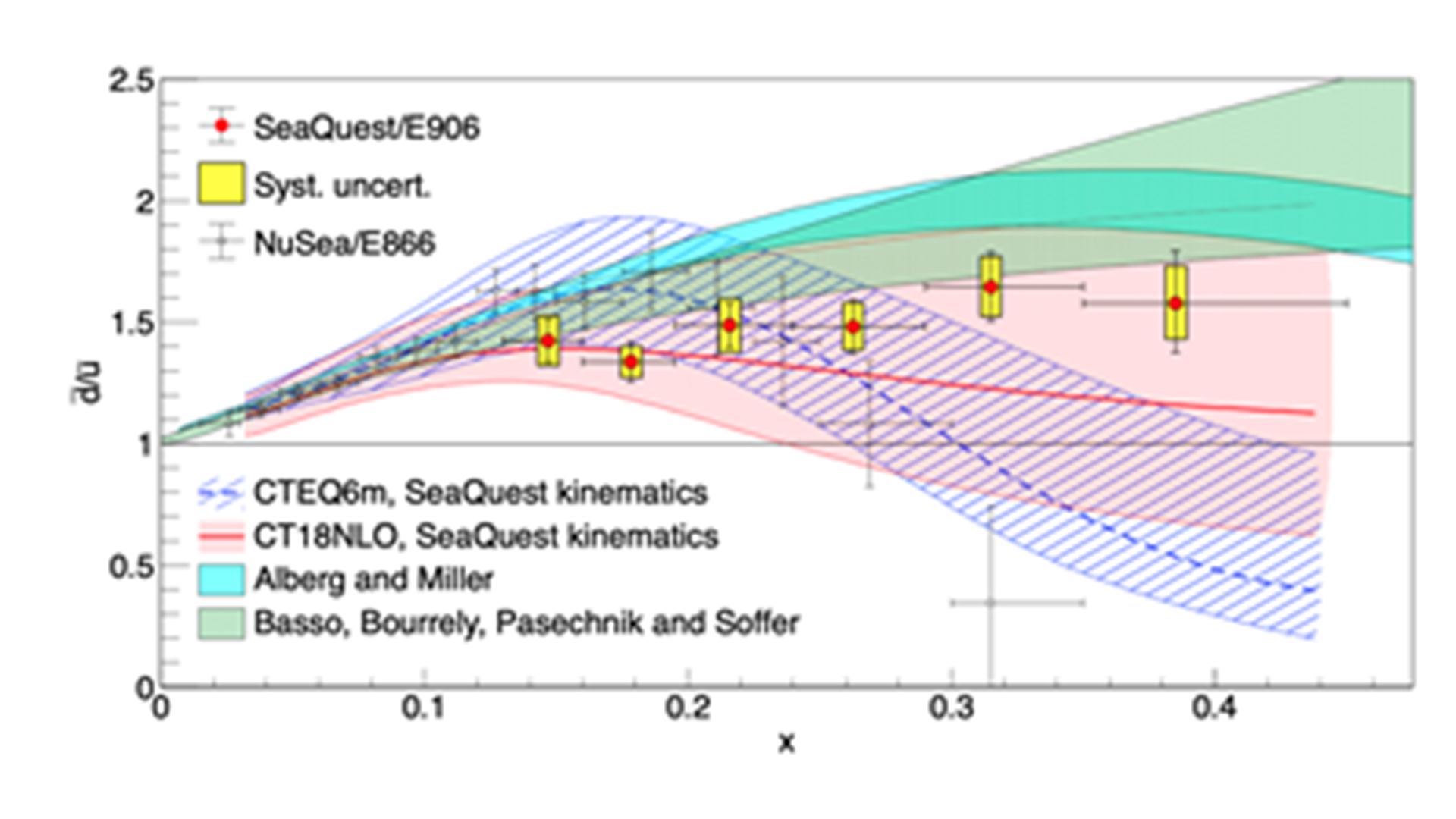
The highest x data points from E866 contain large statistical uncertainties and, so, a new measurement with improved precision for the large x region was warranted. The SeaQuest (Fermilab E906) experiment, using a new spectrometer and a 120 GeV proton beam, recently reported the first result. ² Fig. 2 shows the result from SeaQuest together with the E866. While the SeaQuest result is in qualitative agreement with that of E866, some tension in the d ̅ (x)/ u ̅ (x) ratios at the largest x remains. The SeaQuest result shows that the d ̅ (x)/u ̅ (x) ratios are greater than unity for the entire measured x range.
Implications and prospects
Several models, including the meson cloud model, chiral-quark model, statistical model, chiral-quark soliton model, and instanton model can describe the rise of d ̅ (x)/ u ̅ (x) as x increases in the small x region (see Refs. 3, 4 for reviews). In most of these models a meson cloud is the source of the flavor asymmetry between u ̅ (x) and d ̅ (x).
While the importance of the meson cloud for understanding the nucleon form factors at a relatively low Q² scale is well established, it is interesting that the meson cloud could also lead to a flavor asymmetry in the partonic structures probed at much higher Q² scales. The d ̅ (x)/ u ̅ (x) asymmetry has also been utilised to extract the content of the intrinsic light-quark sea of the proton.4 The flavor asymmetry of the light quark sea has also been explored using lattice QCD techniques.
Several recent global analyses have included the new SeaQuest results in addition to the recent W-boson production data from the STAR collaboration. These new proton PDFs have shown that the SeaQuest data significantly reduce the uncertainties of d ̅ (x)/ u ̅ (x) at large x. The SeaQuest data will place new stringent constraints on future efforts to extract proton parton distributions.
The unexpected finding of the flavor asymmetry of the proton sea has generated much interest and has provided new insights on the origins of the nucleon’s sea. At the Large Hadron Collider (LHC) or the future Electron Ion Collider (EIC), the role of sea quarks becomes more important, since the densities of the sea quarks, especially for the heavy flavors, are expected to rise at the large Q² and small x regions explored in these high-energy colliders. This offers the opportunity to study the flavor structures of the light quark sea at new kinematic regions, as well as the heavy quark sea (s, c, b) of which much less is known.
References
- `Improved measurement of the d ̅ /u ̅ asymmetry in the nucleon sea’, R.S. Towell et al., (E866/NuSea Coll.), Phys. Rev. D65 (2001) 052002.
- `The asymmetry of antimatter in the proton’, J. Dove et al (SeaQuest Coll.), Nature 590 (2021) 561.
- `Flavor asymmetry of light quark in the nucleon sea’, G.T. Garvey and J.C. Peng, Prog. Part. Nucl. Phys. 47 (2001) 203.
- `Flavor structure of the nucleon sea’, W.C. Chang and J.C. Peng, Prog. Part. Nucl. Phys. 79 (2014) 95.
Please note, this article will also appear in the 20th edition of our quarterly publication.