Dr Christine Joblin, from CNRS/Université Paul Sabatier- Toulouse 3, discusses the topic of astro-PAHs, illustrating the potential of laboratory astrophysics to analyse the observations of infrared space missions.
When talking about terrestrial polycyclic aromatic hydrocarbons (PAHs), we have in mind pollutants that are produced by the combustion of waste, wood fires, engine exhausts, or even barbecues. These PAHs present in the gas-phase and at the surface of soot particles are considered as hazardous to health because of their carcinogenic potential. From a different perspective, PAHs are known to be an important component in astrochemistry containing about 10% of the carbon in our Galaxy. These astro-PAHs have the interesting property of strongly glowing in the infrared upon irradiation by ultraviolet (UV) photons from stars. Their emission features are therefore useful tracers of matter evolution in environments irradiated by UV photons, which include galaxies, regions of star and planet formation, and dying stars.
PAHs represent a large population of carbonaceous molecules made of aromatic cycles. They can contain from ten to 100 or more carbon atoms and include many isomers (same mass but different structures), heteroatoms (such as oxygen or nitrogen) and side groups (methylated side groups are common). These chemical specificities are linked to the formation and evolution history of these molecules in their environments. They can be studied in the laboratory using various analytical techniques. However, in the case of astro-PAHs, we have mainly to rely on the analysis of their spectral bands in the mid-infrared, the so-called aromatic infrared bands (AIBs). Only a few of these bands can be observed from the ground, whereas others require observations from above the Earth’s atmosphere. The study of the AIBs is therefore very much related to observations with infrared space telescopes, such as Spitzer (2003-2009; NASA) and AKARI (2006-2011; JAXA) over the last 20 years.
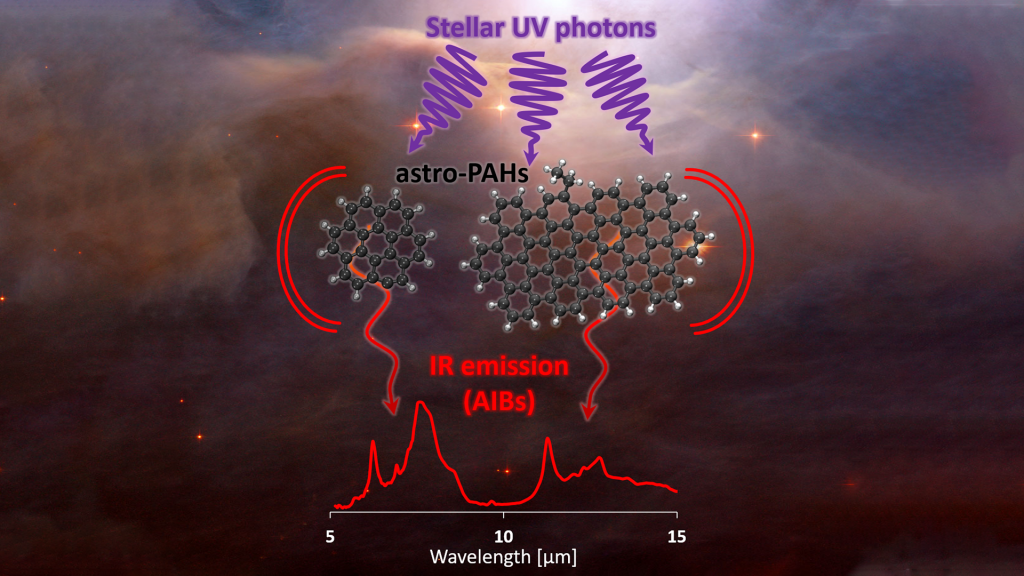
The observational and chemical modelling views: the key role of ultraviolet irradiation
The emission mechanism associated with the AIBs was proposed in the mid-1980s. Upon absorption of a single UV photon, astro-PAHs are heated up to about 1,000 kelvins (or even more depending on their size) and, because they are isolated from their environment, they can cool down to very low temperatures by vibrating in their aromatic carbon-carbon and carbon-hydrogen bonds, which results in infrared photon emission. For years, the PAH model faced some controversy because of our inability to identify individual PAHs. PAH infrared spectra present similarities from one species to the other, and the emitted bands are broad because they arise from hot molecules. It is therefore tricky to disentangle individual contributions. Luckily, it happened that one related molecule, the buckminsterfullerene molecule C60, could be detected in Spitzer observations because it has bands that are significantly shifted relative to those of PAHs. This provided strong support to the PAH model, since C60, which is not a PAH but a cousin aromatic molecule, responds similarly to UV photons. This large molecule is however peculiar by its thermal- and photo-stability, which allows it to survive upon more intense irradiation, i.e., closer to hot stars, relative to PAHs.
Ultraviolet irradiation can change the average charge state of the PAH population, which affects the relative AIB intensities. Since the ionisation ratio between cationic PAHs (PAH+) and neutral PAHs is governed by the competition between ionisation by UV photons and recombination with electrons, the use of chemical models opens the possibility to quantify local physical conditions (UV radiation field intensity and electron density). Ultraviolet irradiation can also lead to chemical processing, which favours the survival of the most resistant species. Chemical models predict that the latter are molecules containing more than typically 50 carbon atoms. We have also more and more evidence that UV processing plays a key role in the formation of PAHs, for which both bottom-up and top-down chemical scenarios are proposed.
The former scenario consists in building up larger species in the gas-phase from small precursors, as it happens, for instance, in hydrocarbon flames. Hydrocarbons and high temperatures can be found in the circumstellar envelopes of evolved stars; the asymptotic giant branch (AGB) phase of low- and intermediate-mass stars is known to contain an active stardust formation zone. There is so far no observational confirmation that PAHs form in this zone. On the other hand, AIB emission is observed only when the AGB star continues its evolution toward the planetary nebula stage in which the central star transforms into a white dwarf that strongly emits in the UV range. The strong variations that are observed in the mid-infrared spectra during this transition point to a complex hydrocarbon chemistry. Chemical models predict this chemistry to be fostered by abundant radicals formed by the interaction with UV photons. However, these models remain limited to describe the growth of PAHs due to the limited available chemical networks.
The knowledge that we have on top-down chemical scenarios is even more partial. The idea is that dust grains can be processed and release PAHs in the gas-phase. Again, the role of UV processing in this chemistry was invoked to account for the evolution of the mid-infrared spectra across the bright interfaces between young massive stars and dark molecular clouds.
The view of laboratory astrophysics
Our current view on astro-PAHs is built on various scenarios that emerged from the analysis of the AIB spectra in relation to the key role played by UV photons in triggering infrared emission and in chemical alteration. These scenarios need, however, to be consolidated and further tested and this cannot be achieved without the contribution of the laboratory astrophysics research field.
First, spectroscopic data on hot PAHs has to be gathered to refine our analysis of the AIBs. Then, all questions related to PAH formation, processing with energetic radiation, and interaction with gas and dust components have to be tackled. Not only laboratory experiments but also numerical simulations based on quantum chemistry are involved in producing data that is crucial to model the infrared emission and chemical evolution of astro-PAHs.
These studies also help us to progress in our understanding of fundamental molecular processes. Experiments have to overcome challenges such as using the right analogues for astro-PAHs (e.g. large versus small species) and mimicking at best the physical and chemical conditions that prevail in astrophysical environments.
When studying the interaction of PAHs with stellar UV photons, one has to consider that astrophysical environments are very rarefied and that some of the processes of interest, such as infrared emission, are slow and involve timescales of seconds and more. To achieve these conditions in the laboratory, it is necessary to couple ultra-high vacuum and cryogenic technologies (very low temperatures below 30K) to isolate PAHs from their environment, with trapping and storage techniques to reach the long timescales. Very few setups can achieve these conditions. These include our PIRENEA setup in Toulouse, which has been specifically developed to study astro-PAHs, and also new generations of cryogenic storage rings such as DESIREE at Stockholm University and the Cryogenic Storage Ring at MPI-K in Heidelberg.
An additional difficulty is that we need access to a large range of energies of the interacting UV photons to mimic stellar irradiation, which requires the use of UV synchrotron beamlines such as DESIRS at SOLEIL. Unfortunately, the cryogenic setups described above cannot be moved at the synchrotron.
Concerning the chemical puzzle of PAH formation, various studies have been performed in a number of groups using reactors such as low-pressure flames, plasma reactors, and laser vaporisation sources. However, none of these sources can mimic the circumstellar environment of evolved stars. This is the reason why a new simulation chamber, the Stardust machine, was built in Madrid (CSIC) as part of the Nanocosmos Synergy project funded by the European Research Council (ERC). This complex ultra-high vacuum machine combines several sources of atoms and diagnosis from surface science to study the formation of tiny dust particles (of the order of 10 nm) in conditions that approach those of AGB stars.
Complementary to Stardust, the AROMA setup was built in our laboratory in Toulouse to perform the molecular analysis of the Stardust samples and provide a census of the families of carbonaceous molecules with very high sensitivity thanks to a two-step laser mass spectrometry. Experiments performed with Stardust and AROMA revealed that the basic chemistry involving atomic and molecular carbon (C, C2) and hydrogen (H2) forms pure carbon clusters and aliphatic carbon species but only very low levels of aromatics.
New experiments are planned to study a more complex chemistry involving silicon and iron in addition of carbon and hydrogen. Using a cold plasma reactor, we have recently shown that the addition of metallic atoms can induce active hydrocarbon chemistry and the formation of metallic nanoparticles.
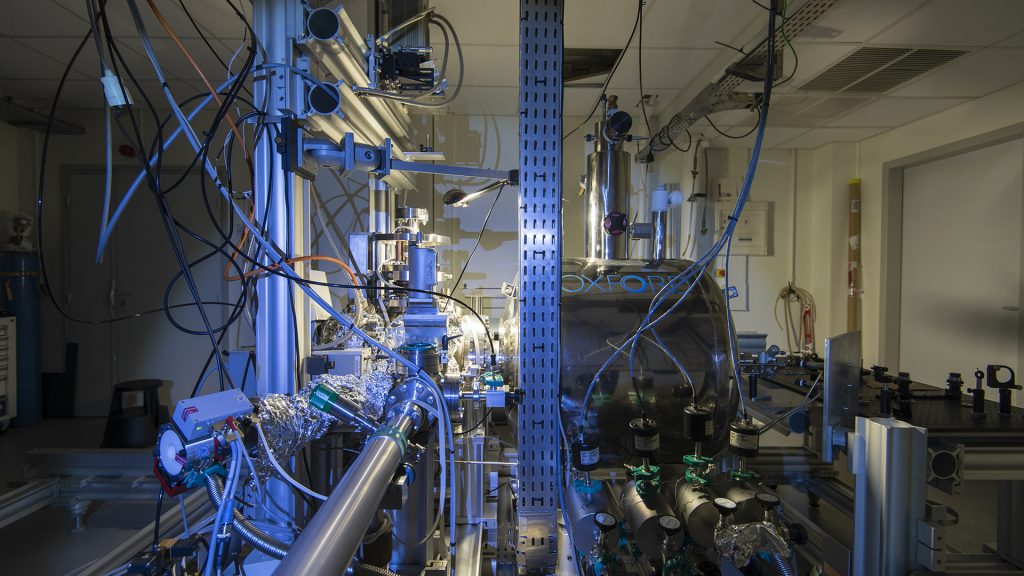
Conclusion and perspectives
The coming years should be strongly marked by the James Webb Space Telescope (JWST, NASA/ESA/CSA) to be launched at the end of 2021. In particular, JWST will provide us unprecedented data on the AIB spectra and their spatial variation in regions submitted to UV photons such as star and planet forming regions. The achieved spatial resolution for AIBs, and in general for dust emission, will be comparable to that of the large interferometer ALMA for molecules, giving us the opportunity to study the interactions between gas and dust. Its unprecedented sensitivity will open the observations of fainter regions that might indeed challenge our view on astro-PAHs. The coming years will also be the opportunity for us to further exploit the complex experimental setups which have been recently developed in the framework of large research projects such as Nanocosmos.
The history of the chemical formation and evolution of astro-PAHs is a particularly complex subject, which is connected to the lifecycle of carbonaceous matter in the galaxy. This topic has now to accommodate new chemical pathways at very low temperatures and without UV irradiation to account for the recent detection by radio observations of abundant small ring molecules in the dark molecular cloud TMC-1 by the Yebes 40m radio telescope and the Green Bank Telescope. Since the TMC-1 region contains prestellar cores that could give birth to stars and planets, it is interesting to relate the matter observed in TMC-1 with that in primitive bodies of the Solar System, comets and asteroids, which preserve some record of the interstellar matter that was composing the presolar nebula. The inventory of PAHs in these bodies, most often performed by meteorite analysis in the laboratory, has now extended to in situ exploration with space probes (e.g., the Rosetta ESA mission) and analysis of material from return-sample missions (e.g., Hayabusa 2 from JAXA). Interesting perspectives are also expected in this field.
Finally, the case of astro-PAHs illustrates very well the potential of laboratory astrophysics (LA), a research field that has grown significantly over the years to support the interpretation of data from space missions and ground-based telescopes. This was made possible thanks to the involvement in this field of a number of physicists and chemists, together with the support of engineer teams, to build innovative laboratory setups. These teams gather a large panel of skills including ultra-high vacuum and cryogenic technologies, ion optics and trapping, laser optics, and mass spectrometry. Whereas the LA community is getting structured and supported around space missions in the US, this is not the case at the European level.
The European community is awaiting a comparable framework as recently discussed at the ECLA2020 international conference, held at the end of September 2021 in Anacapri (Italy). We are therefore hoping that the large dedicated setups that have been developed thanks to consequent research grants will continue to be used in the long term with appropriate support from astronomical projects.
Acknowledgments
This research was supported over the period 2014-2021 by the ERC under the European Union’s Seventh Framework Programme ERC-2013-SyG, Grant Agreement no. 610256, Nanocosmos. This project was developed between CSIC (Madrid) and CNRS (Toulouse), involving three coordinators (J Cernicharo, C Joblin, J A Martín-Gago) as well as contributions from approximately 60 researchers, engineers, and students. The author would like to especially acknowledge the technical team at IRAP/OMP/CNRS, namely A Bonnamy, L Noguès, D Murat, and O Coeur-Joly, for their continuous involvement in developing and maintaining our setups dedicated to laboratory astrophysics.
Further reading (with open access)
1 PAHs and the Universe, C Joblin and A G G M Tielens eds, EAS Publications Series, vol. 46, (2011); www.edp-open.org/images/stories/books/fulldl/eas_46_full.pdf
2 ECLA – European Conference on Laboratory Astrophysics, C Stehlé, C Joblin and L d’Hendecourt eds, EAS Publications Series, vol. 58 (2012) www.eas-journal.org/articles/eas/abs/2012/06/contents/contents.html
3 Nanocosmos ERC Synergy project: nanocosmos.iff.csic.es/
4 Stardust machine: Martínez, L, Santoro, G, Merino, P, et al., Nature Astron. 4 (2020), 97-105; hal.archives-ouvertes.fr/hal-03085475
5 AROMA setup: Sabbah, H, et al., Astrophys. J. 843 (2017), id. 34, 8 pp.; arxiv.org/ftp/arxiv/papers/1705/1705.09974.pdf
6 PIRENEA setup: Marciniak, A, et al., Astron. & Astrophys. 652 (2021), A42, DOI: 10.1051/0004-6361/202140737
7 UV processing of astro-PAHs: Joblin, C, et al., J. Phys.: Conf. Series 1412 (6) (2020), id. 062002, DOI: 10.1088/1742-6596/1412/6/062002
8 (Star)dust formation involving metals: Bérard, R, et al., Front. astron. space sci. 8 (2021), 654879; hal.archives-ouvertes.fr/hal-03227179
9 Observations at the Yebes radio telescope: Cernicharo, J, et al., Astron. & Astrophys. 649 (2021), id.L15, 12 pp.; DOI: 10.1051/0004-6361/202141156
10 PDRs4All: A JWST Early Release Science Program on radiative feedback from massive stars. www.jwst-ism.org
Please note, this article will also appear in the eighth edition of our quarterly publication.