The University of Bonn’s Professor Dr P Martin Sander details his exciting research into fossil bone microstructure and how its findings may produce a deeper understanding of human bone biology.
As Professor Sander puts it, bone was the breakthrough innovation that forged much of life as we know it. Yet, despite being such a crucial anatomical feature, there is still much unknown about the nature of bone – most notably – the conditions that drive fossilisation. Sander’s latest work focuses on plugging these knowledge gaps through cutting-edge analysis of dinosaur bone to understand the enigmatic process of fossilisation and potentially pioneer real-world applications for the medical industry. This research is part of a large coordinated research project funded by the German Research Foundation; funding is gratefully acknowledged.
The Innovation News Network spoke to Professor Sander to find out more about bone microstructure.
What is special about petrified bones?
Unlike many other fossils, bone will almost always preserve not only its shape but also its microstructure, specifically the histology. Histology is the microstructure at the tissue level. In studying organisms, there are various levels. You start with the shape or morphology, the macro level, the micro level, the ultrastructure, and finally, the nanostructure. This poses two fundamental questions: why is there fossil bone, and why does it preserve its microstructure?
We know about dinosaurs because we have their fossilised bones. But why does bone fossilise? Because it shouldn’t. These questions have become urgent. The problem is nobody’s ever worried about it – everybody’s just always taken it for granted.
Interestingly, if you take petrified wood or a fossil clam, you cannot be guaranteed to see any microstructure. A lot of fossil wood, for example, from the Jurassic, is beautiful, but you won’t see anything in the microscope. Similarly, with fossil shells, you’re unlikely to see much of the original structure of the fossil shell. However, in bones, it’s the other way around. You’re highly unlikely not to see the microstructure.
How do fossil bones provide more than shape information?
Because they have that microstructure which gives us an immediate and direct comparison between what we would see in a modern animal, say a crocodile or a bird or a human, and the dinosaur. This basic fact has been known for 200 years, but only in the last 40 or 50 years have people started making sense of it. For example, you can see growth marks in dinosaur bones that explain how old the dinosaur was when it died. If you do this for many dinosaurs and many species, you see patterns that are related to the ecology and evolution of dinosaurs.
The image below shows what fossil dinosaur bone looks like under the microscope. The dark areas are where the blood vessels were in the bone, and you see growth marks. Like in a tree, you get a growth record. By analysing growth marks, we can age the animals.
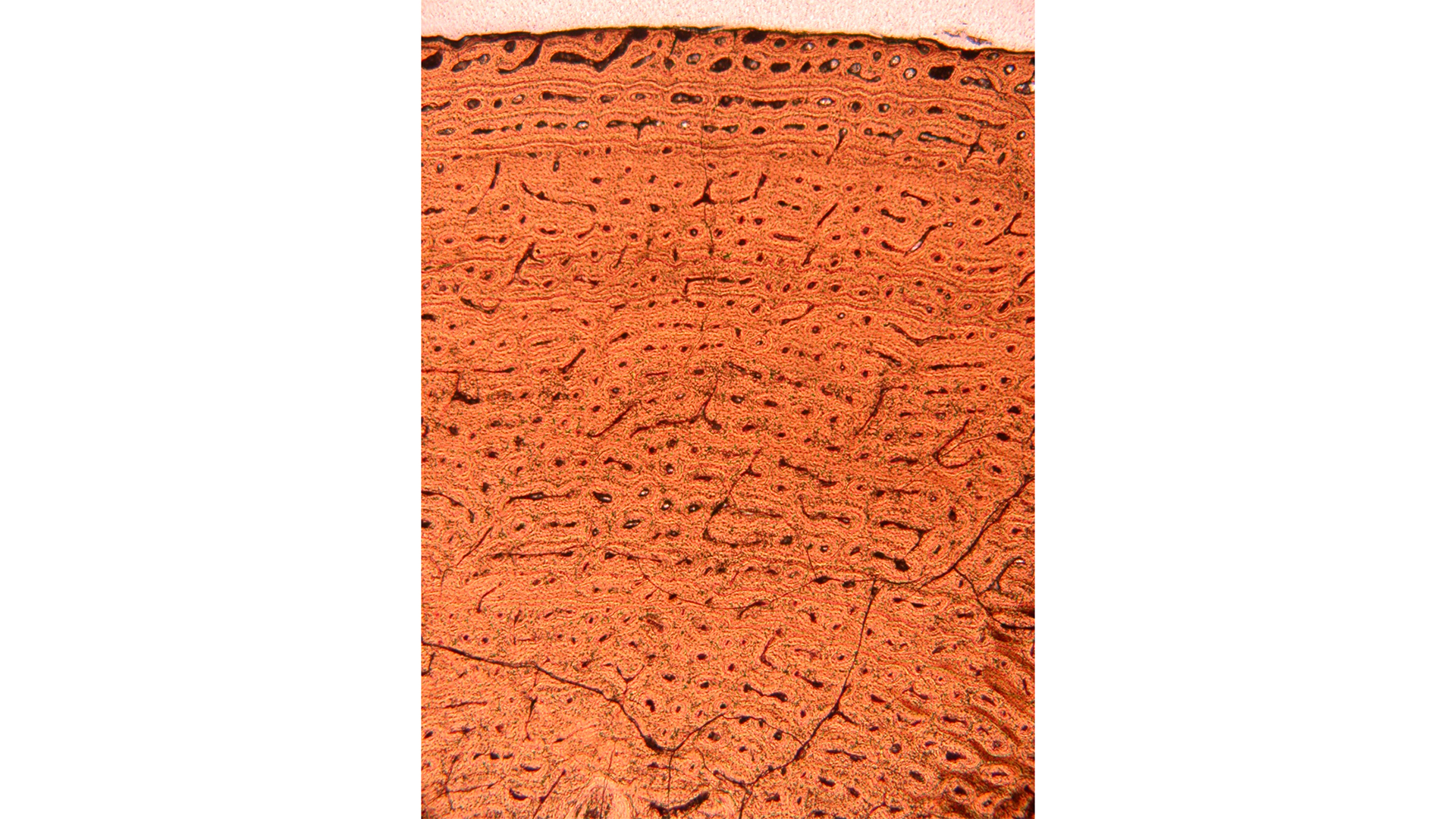
This example here is particularly striking. This was an animal we named Europasaurus – a type of sauropod that lived 150 million years ago. It is the first proof that the giant long-necked sauropods underwent a process known as island dwarfing. Essentially, if you were to go to any larger Mediterranean island (Crete, Sicily, Malta, etc.) around 50,000 or 100,000 years ago, more likely than not, you would have seen dwarf elephants that got onto the island as normal-sized elephants but then responded to the limited resources on the island by getting smaller and smaller with each generation, until they reached an optimal size, as little as a donkey. With this sample, we could tell that dinosaurs also underwent this evolutionary process of island dwarfing.
What can we learn about living animals today from bone microstructure?
The image below is from a plesiosaur – one of the first sea monsters to be recognised. You see a different illumination in the light microscope, to observe the bone histology. The pink, blue and yellowish stuff is the bone matrix. This specimen is 205 million years old, and the only way you know it’s a fossil is that there is an infill of minerals in the original spaces where the blood vessels were. If you zoom in on this, you see mineral growth. You wouldn’t see that in a modern bone. Additionally, all the black things you see are bone cells; the more bone cells you have, the more metabolically active you are. Here you have a bone with more bone cells than bone matrix in some places, which suggests crazy fast bone tissue formation.
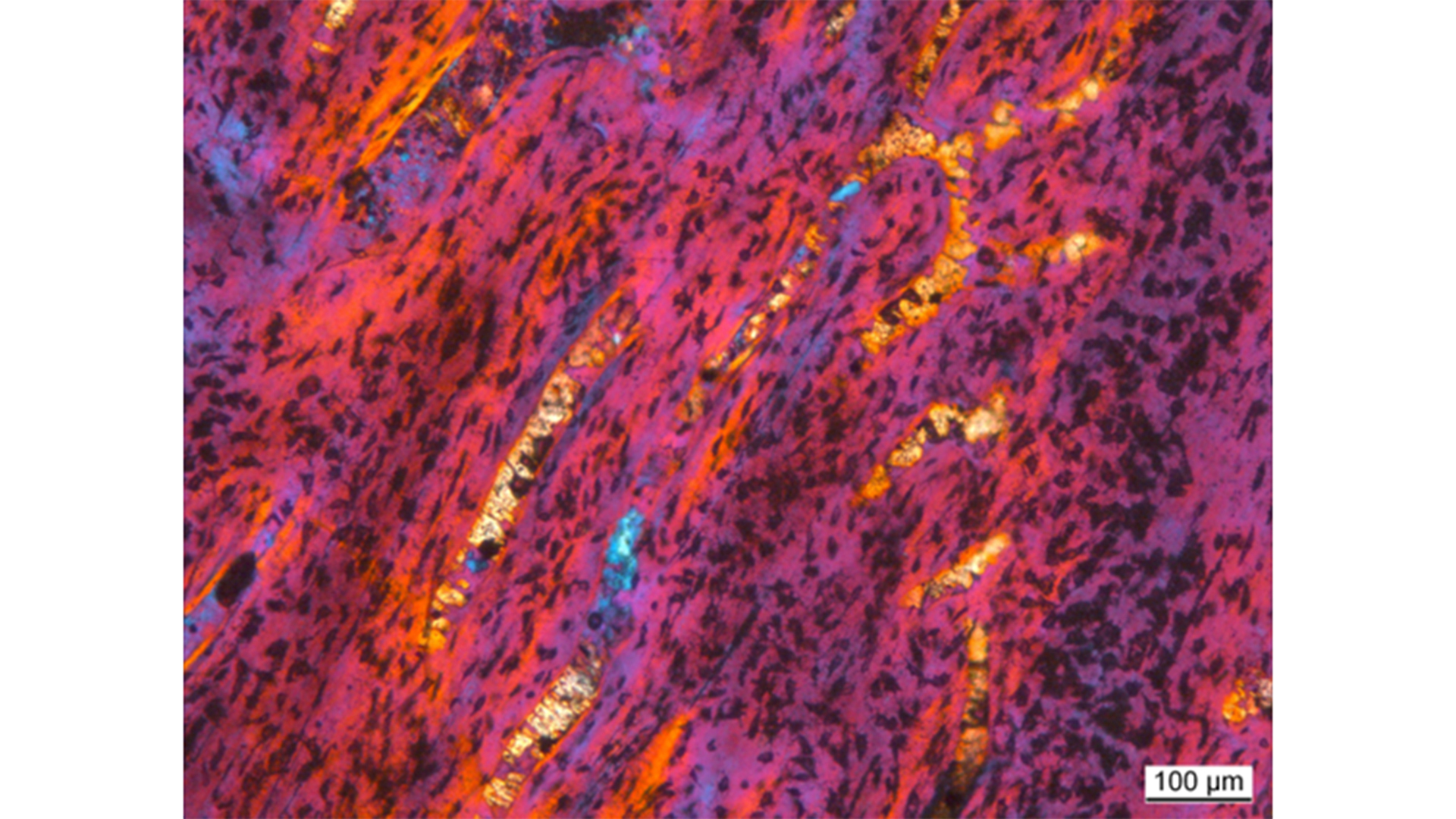
Therefore, this plesiosaur was growing crazy fast. We can see how they did this by looking at modern animals. For example, birds grow crazy fast, not as large, but crazy fast. Because fast growth requires a rapid metabolism, this image tells us immediately that plesiosaurs were warm-blooded.
How do you study fossil bone microstructure?
That’s the ugly part. Most of the time, you have to cut up the bone. If you go to a museum and there is a dinosaur skeleton, and you ask the curator, can I have a slice of that? The curator is not going to be very happy. So I came up with this device shown below that can perform a biopsy of a dinosaur bone.
The method works by taking a controlled sample, always in the same location. In this case, a smallish dinosaur bone. This device is a household electric drill, which has a diamond-studded coring bit. Ideally, to study fossil bone histology, you want to saw right through that bone, but that makes the curator unhappy. So to make the curator less unhappy, I’ve devised this biopsy method where we take a small core sample which then is processed into a thin slice of bone called a “petrographic thin section”, about the 20th of a millimetre – 50 micrometres thick. This is so thin that the fossil bone becomes translucent and we can study it under the microscope.
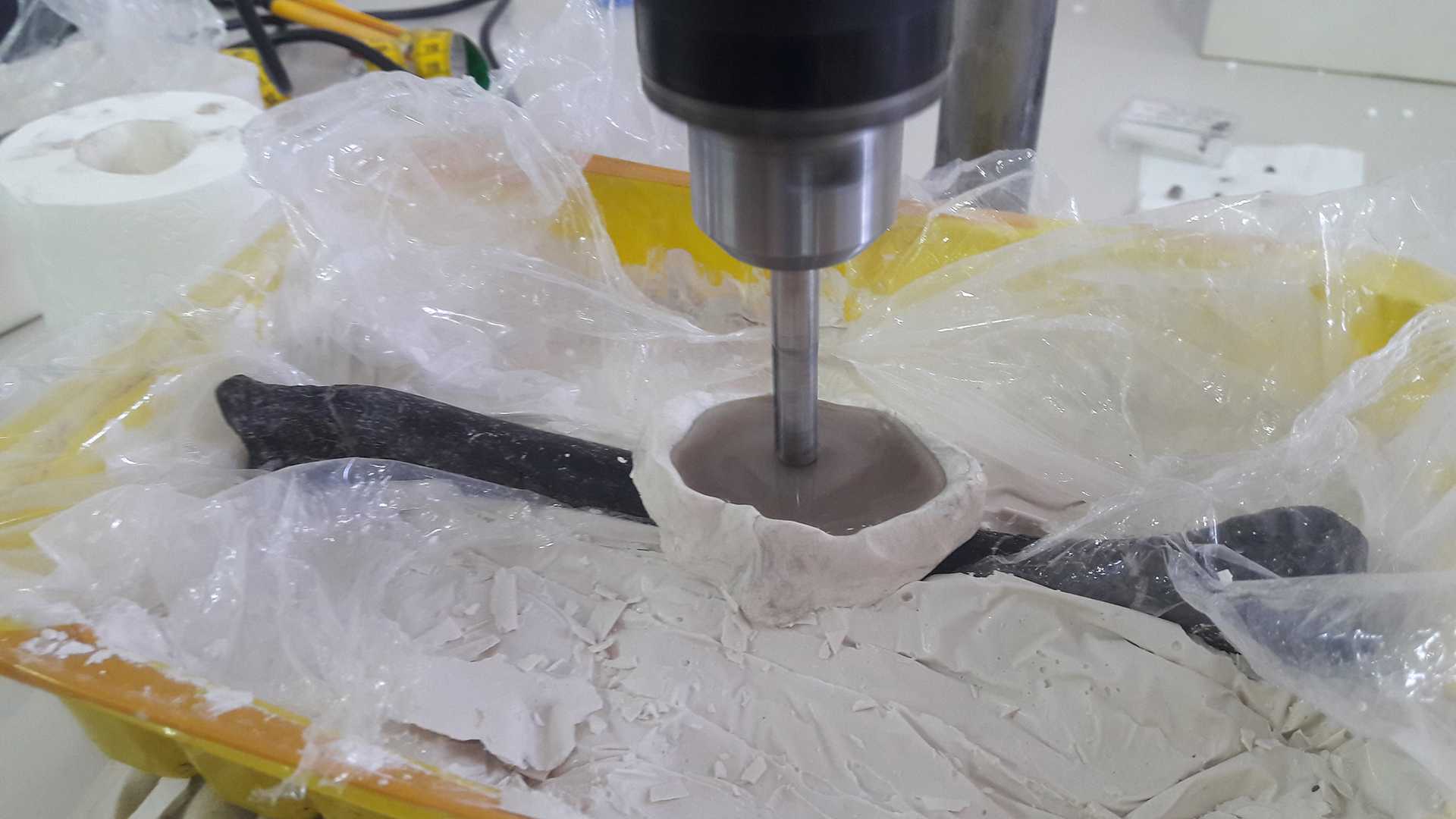
The images below show petrographic thin sections, a technology around 200 years old, lying on the table next to the microscope. The technique of slicing rocks and fossils into skinny slices may be old, but the interpretation is new. Once we’ve ground a thin section, we can see all these structures and cells, which is the same thing the pathology lab will do. For example, the pathologist will take a biopsy if you have bone cancer. They will look at the thinly sliced tissue under the microscope, and we’re doing the same thing, except the technique is different because fossil bone is rock, after all, so we have to grind and cannot cut it with a microtome knife.
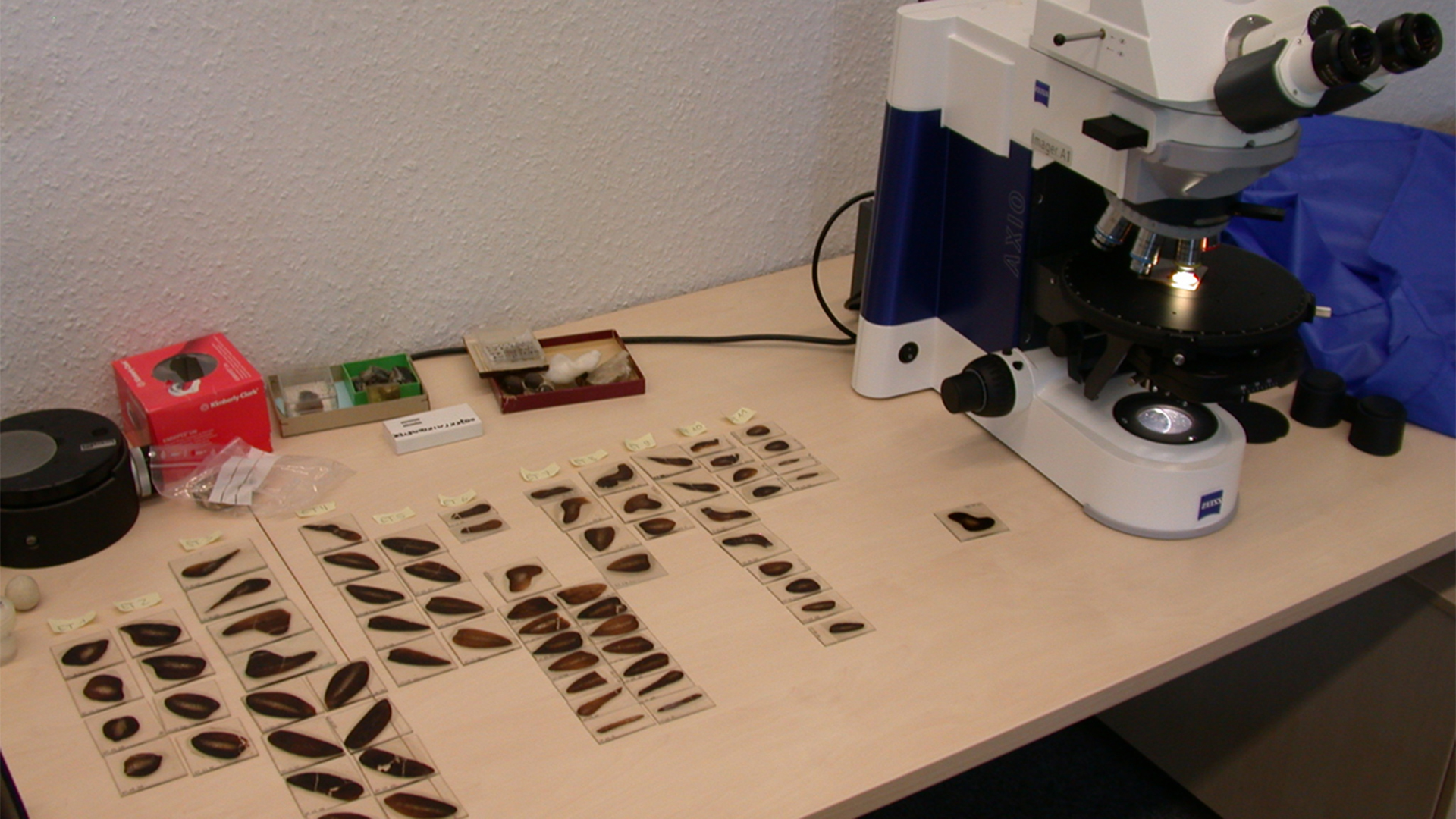
Why do dinosaur bones fossilise?
That’s the million-dollar question. Basically, in rare instances, you only get the shape. For example, in Australia, plesiosaurs are occasionally preserved as opal. So there you have a plesiosaur skeleton worth millions of dollars because it’s made of precious opal. But for our purposes, that fossil is worthless because only the shape of the bone is there. What happened is that the bone was covered by sand, and then the sand turned into stone, and then the bone material got dissolved away. The resulting hollow spaces were filled with precious opal. But this kind of preservation is the big exception. The rule is that the microstructure is preserved. That is the huge problem we’re facing right now, but why? Because bone is a mineralised tissue, meaning most of its volume is mineral, specifically a mineral called apatite, which is simply calcium phosphate. But the mineral is embedded into organic material, specifically collagen.
Collagen is the organic component of bone. The bone matrix consists of these fibrils, which we also found in fossils. These fibrils contain 30% collagen and 70% apatite mineral in the living bone and then they get petrified, losing all the collagen. Fossilisation is like Medusa’s gaze. It looks at the bone and turns it to stone. What has to happen is that without compromising the microstructure, all the collagen has to go away and be replaced by mineral. This is extremely strange because if you have this replacement of 30% by volume of material, you would think that you get a loss of information, but you don’t. Loss of information is precisely what is happening in the other kinds of fossils, such as in wood or shells. Through the fossilisation process, we get a lot of material exchange – an extreme case would be that opalised plesiosaur.
So we know there has to be a massive exchange of materials, but we don’t know how. The added twist is that despite replacing 30% of the material, the fossil bone is still apatite, so it’s again calcium phosphate, but with a slightly different composition because during fossilisation, the bone takes up fluorine from the groundwater. This is why we have fluorine in our toothpaste because this element binds to our tooth enamel, which is also apatite, and strengthens it. As the collagen is replaced by apatite during the fossilisation process, you also have to add phosphorus because there’s almost no phosphorus in the collagen. So the phosphorus has to come from somewhere because the fossil bone is 98% apatite. That means we must make this new apatite and need phosphorus for that. If you think about phosphorus, it is not just floating around in the environment. Phosphorus is always something that is rare and is particularly rare near the surface because plants like it.
Plants will take up the phosphorus and not give it away. So one of several questions we don’t understand is where all the phosphorus comes from in fossilisation, and then we start to think about the environment. So we have the animal; when it dies, that is the beginning of fossilisation. We call this taphonomy – the processes between the death of the animal and when its remains enter the lithosphere, so they become buried by sediment.
Then the next strange thing happens – the fossilisation process is independent of the environment. So if an animal dies in the desert, its bones can fossilise just as much as when a whale dies in the open ocean. So there seemingly is no preferred environment for bone to fossilise. For example, you might think that if an animal carcass is buried in the stinking mud (it’s stinking because there is no oxygen) at the bottom of a pond, you will get better preservation of bone. That is not the case. So most big dinosaur bones from North America are all in river sediments.
Specifically, you could hypothesise that the mud at the pond or ocean bottom, where we have little oxygen, would have fewer or only special kinds of bacteria and no scavengers, so we get good bone preservation. But that is not so. We get good preservation of the entire animal. So the bones won’t get scattered. We might get some soft parts preserved and so on. But the bone of such a carcass is not any better preserved than an isolated bone in a river channel. Bone seemingly does not care in which environment it fossilises, and this is a big puzzle. What this means to me is that it is an intrinsic process. When the animal dies, the bone dies. Bone is a living tissue, which is one of the cool things about it because it’s full of living cells. Those cells die as the whole organism dies, and fossilisation must start.
How long does this process take?
It really must be pretty fast. That’s the bottom line. Usually, when a person dies and is buried, the chances of fossilising are fairly low because the corpse decays. Therefore, a bone in a graveyard will not fossilise. Skeletons will last maybe 10,000 years, but typically only for a few hundred years, and then they’re gone. But then the question is, where do the fossils come from? Because when we examine deposits that are maybe 20,000 years old, we start seeing well-preserved fossilised bones alongside very poorly preserved bones that are nearly gone. But once we get to around two million years ago, every bone that’s two million years and older will be well-preserved at the microstructural level.
I think what’s happening is that early on, maybe within hours of death, it’s decided which way that bone will go, towards fossilisation on one side or destruction on the other. If such short time frames are involved, then this might be amenable to experiments. We’ve been trying experiments to fossilise bone, but nobody’s been able to do this very well. So the time frame could be between days and ten thousand years. But it seems that if you’re not fossil by 10,000 years, then that’s it; you’ve lost your chance.
Where do dinosaur bones get their colour in the microscope from?
The image in Fig. 2 is in cross-polarised light, and the colours tell you about two things. First, there are still bone fibrils. In this case, we can’t see the ultrastructure. We only see the microstructure or histology because we see the bone cells that are part of the microstructure – the dark blobs, which all have little things sticking out. That’s the cell or cell space, and we can even go as far as to get these 300 million-year-old bone cells out of the bone.
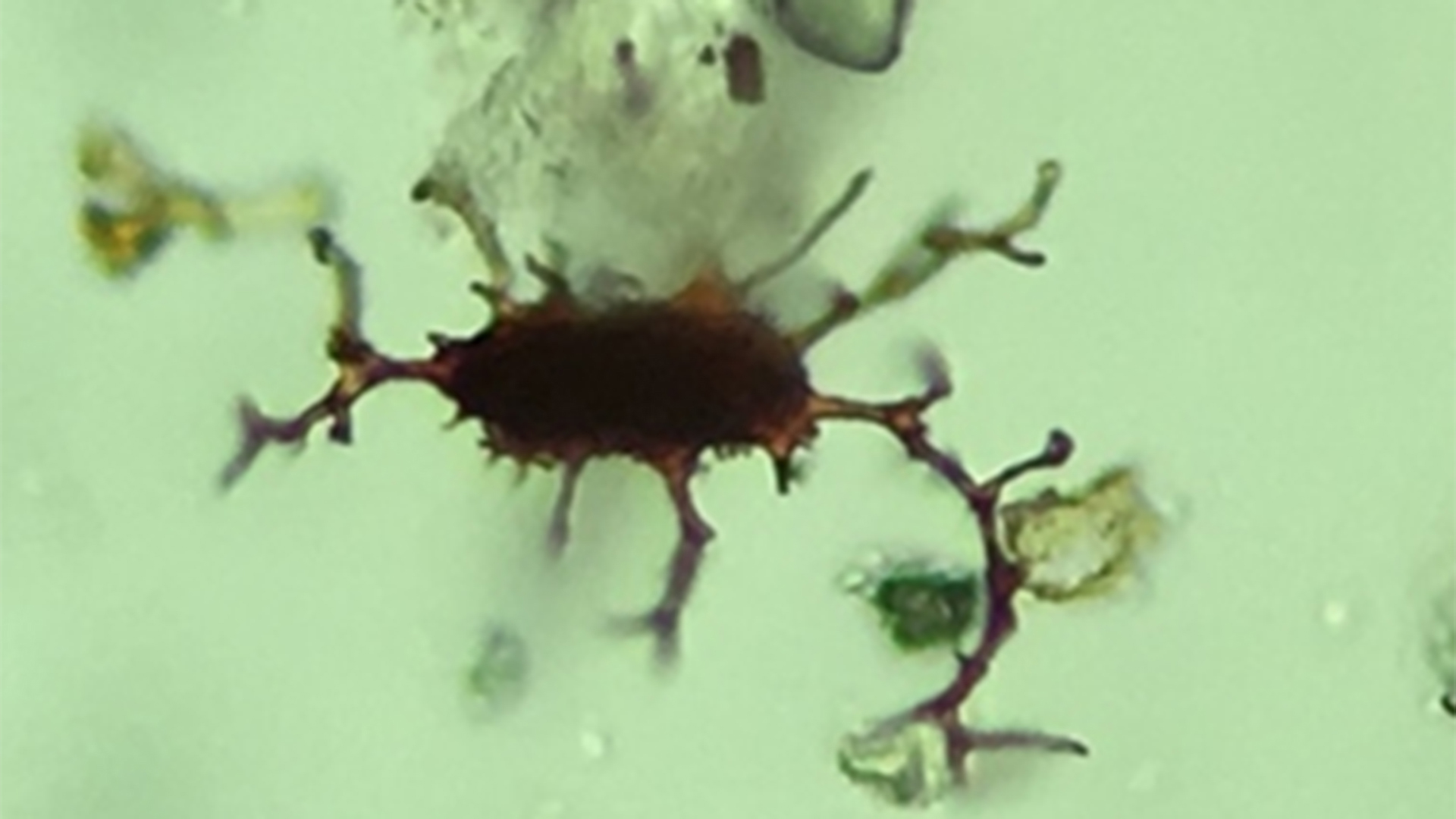
We don’t know what they are, they’re not living anymore, but they’re some kind of fossil bone cell, and in between, you see crazy, psychedelic colours produced by birefringence in the bone. This signifies that bone fibrils are preserved, as in almost all fossil bone.
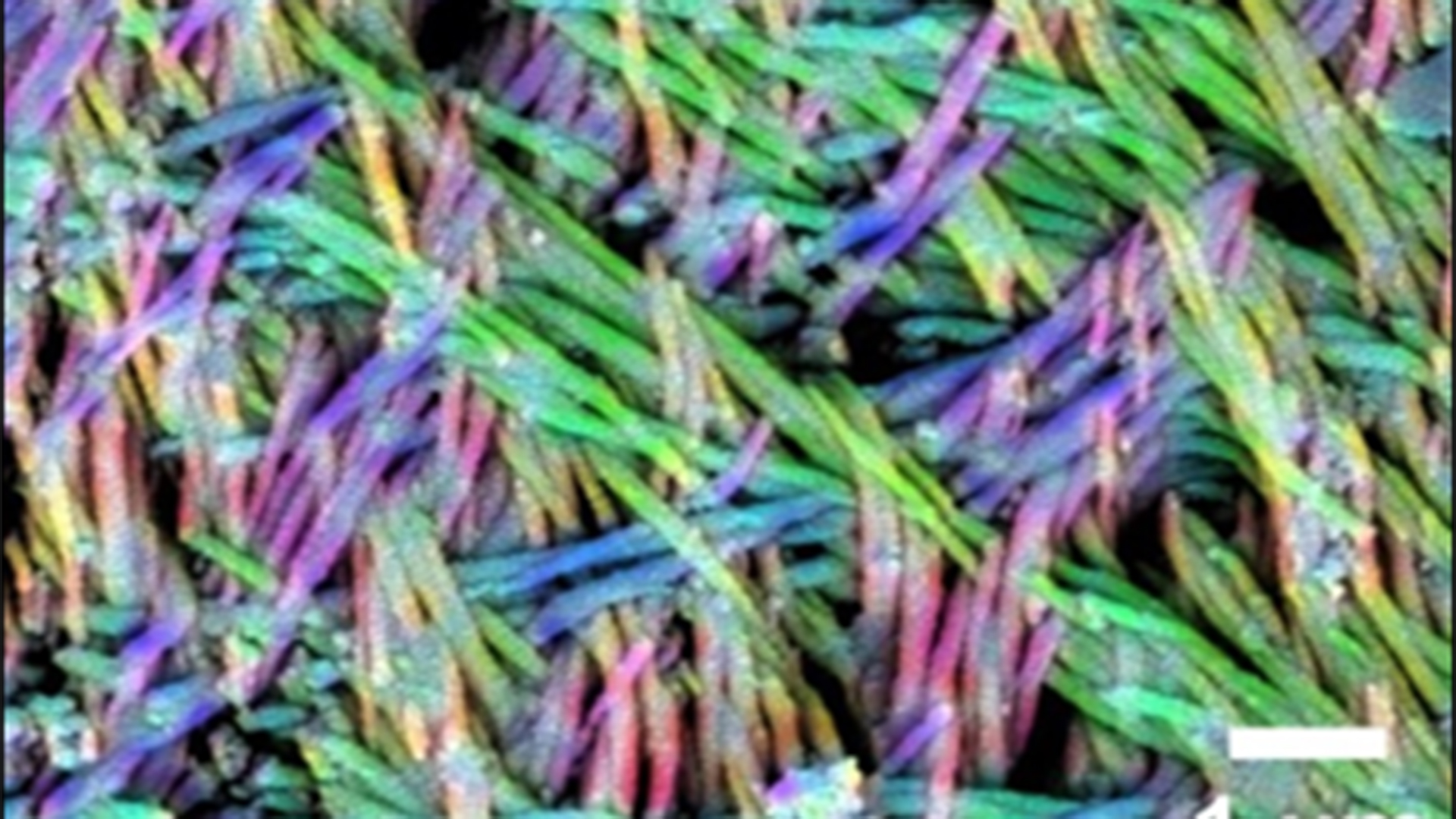
The image in Fig. 6 was produced by a French citizen scientist using scanning electron microscopy (SEM), and I nearly fell off my chair when I saw this first. We are looking at an internal surface bordering on the bone marrow of a 150 million-year-old Jurassic ichthyosaur bone, and you see all these little strings that are all bone fibrils. The colour was added after the image was taken, and all fibrils of the same direction have the same colour.
The collagen is gone, but the fibrils are still there. This explains why in the light microscope images (Fig. 2), we still get the colourful birefringence. In this figure (Fig. 6), we can see the direct preservation of the microstructure as bone fibrils.
At first, we thought this was the great exception, but now we’re beginning to think it is the rule, and we don’t know why. After discovering this, we contacted a leading British expert on bone biology in London. He also nearly fell off his chair when he saw the SEM image in Fig. 6 because he had made a career of producing images of this kind in living bone in horses and humans and so on.
So not only is the microstructure preserved, but we get, in many cases, the ultrastructure preserved. But again, we don’t know why.
What wouldn’t we know if bone would not fossilise?
If their bone had not been fossilised, we would know preciously little about vertebrate animals in the geological past. We wouldn’t know that there were dinosaurs. We would just have impressions of fossil fish, a few dinosaur bones, etc. If we only had the shape, then we could have nice dinosaur skeletons, but to really understand the biology, we need the microstructure in addition to the shape of the bones, and this has really been a revolution in palaeontology. The microstructure ties in so closely with what we can see in modern bone.
We can have vertebrates without bone, like a lamprey, that has no bone. But humans are the way we are because we have a bony skeleton. But what do I need a skeleton for? If you’re in the water, you need a skeleton to attach muscles to, like a clam – a clam shell opens and shuts because the shell is the skeleton and the muscles operate the shell. Once you go on land, you need a skeleton to support yourself, and that skeleton is called wood in trees, and in animals, it’s bone. The cool thing about bone is that it can change shape as it grows, something that clam or snail shells and insects and trees cannot do. The way this works is that bone can change its shape from the inside because there is a process called remodelling that will take away old bone and put it in new places to grow complex shapes that no other animal or plant can. This is why we vertebrates are so hugely successful.
What is special about bone as a biological material?
Because bone preserves not only the shape but also the microstructure, we have this direct ability to compare at the micro level with living things. One thing I am thinking about with my colleagues in this microstructure and ultrastructure project is maybe we can test hypotheses from medicine by studying fossil bone. If you study living bone tissue, you always run up against one problem: bone is a mix of mineralised and unmineralised parts, and studying those together is extremely difficult. You can take the mineral away, but then you’d lose the bone fibrils. You can see the cells. Or you can take the cells away, but then you only see the mineral. But fossilisation has possibly solved this problem by mineralising the soft parts. It could be we’re getting to that point where we can use palaeontology to advance medicine because some bone-building processes are poorly understood, and we’re close to understanding them through studying fossils.
Additionally, no other material is as good as bone at reacting to loading. If you have a tree branch that sticks out, the tree will put more wood to make that branch stable. But in bone, a lot more happens than that, and that’s a big problem in medicine, too, especially for astronauts in space. If you don’t load the skeleton like in space, or because you cannot leave your bed, your bone gets resorbed. And if you break a foot, then that changes the load on your leg and your whole skeleton will react and get out of whack because bone reacts to loading. This also means that if you often lift heavily, like only with one arm, then this will show up in your bones because the bone will adapt in the living organism.
Why is a bone skeleton not made of another material like calcium carbonate or wood?
There is no good answer, except some of it is history. I think calcium carbonate has one big disadvantage as biomineral because it is very soluble. So that means as an organism, you have the advantage that calcium and carbonate ions are super easily available everywhere, and phosphate ions are rare, but calcium phosphate (apatite) is more stable to dissolution.
Then you could say, well, what about wood? Well, wood is pretty good, but the problem is that it is mostly dead tissue, although it still conducts water. But there are no living cells inside wood, and of course, you could imagine that evolution would have found a solution to keep wood alive and make it able to change shape, but it hasn’t happened. But in terms of animals, it seems to be a trade-off. Phosphorus is difficult to get a hold of, but because apatite is less soluble and offers the advantages of durability, it is a successful option. But we don’t really know.