A collaboration of engineers, biomedical scientists, and clinicians in the Netherlands has recently reached a breakthrough in cell-based bone regenerative therapies.
Although bone has a great regenerative capacity, up to 10% of defects cannot heal without intervention. As a result, millions of surgeries are performed to restore bone defects each year. These defects can have a congenital, traumatic, infectious, or oncological cause. For over a century, the gold standard clinical procedure in orthopaedic and maxillofacial surgery to restore such defects has been the transplantation of bone harvested from elsewhere in the patient. This leads to the creation of a second surgical site for the patient, which is often associated with complications such as donor site morbidity and pain, as well as an extended duration of hospitalisation.
To overcome the additional burden on both the patient and society, various alternatives have been proposed over the years, but none have shown the potential to become the new clinical standard.
Regenerative medicine and tissue engineering are fields that have been addressing this demand for bone substitutes in recent decades. Classical approaches aimed to mimic intramembranous (direct) ossification by utilising biomaterials, e.g., those that resemble the bone’s inorganic calcium phosphate component (hydroxyapatite), either alone or in combination with stem cells from the mesenchymal lineage (multipotent mesenchymal stem or stromal cells – MSCs – are typically isolated from the bone marrow and used in bone regeneration). Though effective to some extent, regeneration of large defects is still beyond our reach.
Endochondral ossification
More recently, the field has adopted the body’s indirect mechanism for bone formation: endochondral ossification. In this process, bone is formed from a cartilage template, paralleling the process that naturally takes place in the growth plate. The cartilage cells named ‘chondrocytes’ continue maturation towards a hypertrophic (swollen) state. Then they produce an extracellular matrix with hydroxyapatite and factors that attract progenitor cells and induce blood vessel invasion and tissue remodelling cells such as osteoclasts. Together, through a cascade of events, these cells eventually convert the hypertrophic cartilage tissue into bone.
When recapitulating this approach in the lab, the MSCs are stimulated to form a hypertrophic cartilage tissue. Once such constructs are implanted in animal models, they are remodelled into host-derived bone. This principle was shown effective in (partially) restoring bone defects but also shown to be sufficiently effective to form bone where normally no bone is grown (osteoinductive at ectopic locations), i.e., under the skin.
This approach offers several advantages over conventional clinical solutions or alternatives: the implanted constructs completely remodel into patient-own bone, it is possible to use cells from a donor, which eliminates the need for tissue harvest from the patient, and the constructs are highly osteoinductive, which means they provide strong cues for bone formation.
A novel approach
A recent breakthrough was accomplished in the Regenerative Medicine Center of the University Medical Center in Utrecht, the Netherlands. A novel approach to developing a hypertrophic cartilage construct displayed an unprecedented osteoinductive potential in vivo.
Following implantation into a critical size (i.e., will not heal without intervention) long bone defect, the voids were fully bridged after just FOUR weeks and remodelled into mature bone just a few weeks later.
Additional remarkable features of this invention include the option to use donor-derived (allogeneic) cells and the fact that the cells within the implanted constructs were killed before implantation. Together, the donor-derived origin and dead cells in the constructs open-up the avenue for clinical implementation, as it avoids the need for harvesting cells from patients and offers the potential for an off the shelf product. More specifically, cells can be harvested from, for example, bone marrow of donors or surgical waste materials and expanded in the lab to generate sufficient cells for treating many patients. Furthermore, the dead status of the tissue upon implantation enables storage of the implants, which eases distribution and logistics.
In addition, their new method can be used to circumvent an important obstacle in upscaling the size of engineered tissues to the centimetre scale. Thus far, the upscaling of tissue engineered constructs has been hindered by the lack of functional vascular network, which leads to cell death in the centre of large constructs. However, since the cartilage constructs are actually dead before implantation, it is possible to first generate small tissue building blocks in the lab that, after killing, can be assembled into larger structures. By adopting such modular approaches, complex implant shapes can be acquired, especially when combined with recent advances in the biofabrication and 3D printing field (see Fig. 2 below).
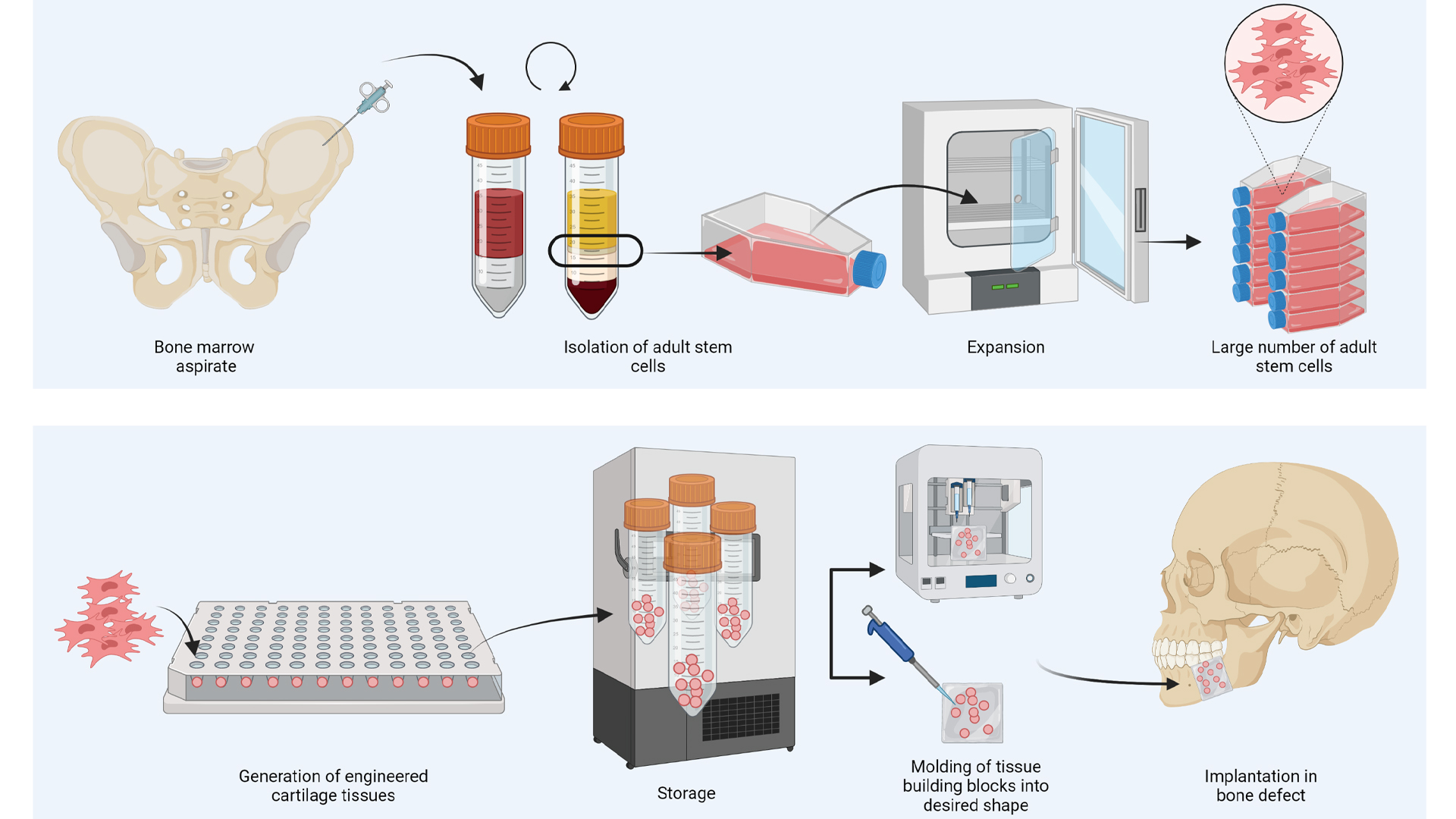
Hot topics in vascularisation
Besides the endochondral focus, the Gawlitta lab is focusing on the regeneration of large tissue constructs with a pre-fabricated vascular structure to support and accelerate the formation of bone tissue. Hot topics in this area include the interconnection of vasculature on multiple scales, simultaneous target tissue formation, functional perfusion, and anastomosis.
To elaborate, microvessels or capillaries can self-assemble in small bone constructs in the lab. Typically, these microvascular networks form by co-culture of at least two cell types. However, connection of such microvessels to larger collecting vessels is key to building a vascular tree. Currently, cell-based approaches to vascularisation are limited to either macro- or micro-vascular structures alone. To adequately perfuse a tissue to provide nutrients and drive maturation of cells within a tissue engineered construct, there is a need to mimic the hierarchical branching structures of vessels. To bridge this gap, the Gawlitta lab is focusing on various strategies to interconnect multi-scale vasculature and on in vivo surgical approaches that ensure immediate anastomosis to the host circulation and perfusion of implanted pre-vascularised tissues.
Future endeavours
The Dutch research team is currently focusing on studies that will elucidate the mechanisms underlying the accelerated bone regeneration and on preclinical studies that will pave the way for a first-in-man clinical trial. A key aspect herein is assembling interdisciplinary teams to tackle the diverse challenges ahead. These will consist of the scale-up of cell expansion and the automated formation of cartilage tissues in the lab, the conversion of methods to GMP-compliant variants, and the exploration of restoration potential in clinically relevant bone defect models.
The research is embedded in the Regenerative Medicine Center Utrecht, where basic science and emerging technologies are integrated with patient needs and the infrastructure, knowledge and creativity are present to convert an idea into a tangible benefit for patients. Over 300 scientists are housed together in this dynamic hub from biomaterials, engineering, biomedical, and clinical backgrounds. Cross-fertilisation of their expertise leads to innovative clinical translational therapies. The GMP-accredited Cell Therapy Facility supports the development of lab findings to clinically applicable products. The on-campus and in-house spin-off incubators and patent office assist in the realisation of new patient therapies.