Physicists from Syracuse University, working at the CERN Large Hadron Collider (LHC), discuss their involvement in the LHCb experiment and the search for evidence of physical forces beyond those we know, called ‘New Physics’ (NP).
In elementary particle physics, we deal with the smallest known basic elements of matter, quarks and leptons (shown in Fig. 1). The quarks feel all known forces: gravity, electromagnetism, weak (for example radioactive decay), and strong (for example nuclei formation). It takes three quarks to make a proton or a neutron, or other baryons, and a pairing of a quark and an anti-quark to make a meson.
The most common lepton is the electron (e−). It has two heavier duplicates, the µ− and the τ−, which are not stable and decay via the weak force. This triplication pattern also holds for the quarks. For each charged lepton there are also three associated almost massless neutral leptons called neutrinos.
The quarks and leptons are treated by theory as particles with mass but no size. This seems nonsensical, but so far the theory has worked (perhaps because they are very small; the upper limit on the size of the electron is 10−18 meters; for comparison, the proton is about 1,000 times larger). However, the theory cannot predict the masses of the quarks and leptons.1
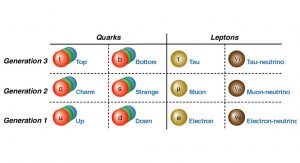
Another mystery is how the Universe evolved from the primordial ‘big bang’2 that had equal amounts of matter and anti-matter to the current matter dominated one. A necessary ingredient is ‘CP’ violation, a phenomenon where particle decays are not the same as those of the anti-particle.3 This phenomena has been observed, but it appears to be too small to account for the particle-antiparticle asymmetry we now see. Furthermore, we know from observations of how galaxies rotate that there is a fog of matter scattered throughout the Universe that does not emit light, and so is called ‘dark matter’, but we know little else about it and would love to produce it in our experiments.4
CERN, the European Organisation for Nuclear Research, is currently the world’s leading center for elementary particle research and home to physicists from all continents, except unpopulated Antarctica. The world’ highest energy accelerator, the Large Hadron Collider (LHC), constructed at CERN, collides proton bunches with an energy of 6.5 1012 eV head on every 40 10−9 seconds. An aerial view is shown in Fig. 2. The interacting protons produce a slew of many particles, generally hundreds in each collision. It is our job to find the interesting ones.
In 2012, the Higgs boson, which had been predicted, was discovered as having a mass about 134 times that of the proton. This was the last particle that fit within what we call the ‘Standard Model’. However, this paradigm cannot explain the quark and lepton masses, or dark matter, and other interesting but detailed observations. Searches for other particles of similar or much larger mass have turned up empty so far. This is consequential since some theories of ‘New Physics’ (NP) had predicted new ‘supersymmetric’ particles, where the known particles were duplicated with different properties but much higher masses. So, the hunt for NP has expanded.
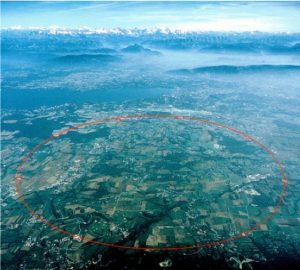
The LHCb experiment
While the ATLAS and CMS experiments at the LHC seek to find directly produced new heavy particles which are symptomatic of new forces, the LHCb experiment looks for indirect evidence by examining b and c quarks. These quarks are not stable. The have a lifetime of ~10−12 seconds. This is very short, but because they are moving so fast in LHC collisions they travel about 1cm before they decay into other particles. This is long enough for us to dig them out from the other particles produced in the proton-proton collision.
To do this, LHCb has a device called a ‘vertex locator’ that can measure the ionisation of the particles as they pass through the silicon in the detector and thus ‘see’ the decays.5 The crucial point is that any new heavy particles influence the decays and we can see evidence for them at much higher masses than the LHC can directly produce. We can also measure CP violation in many ways. Thus far, we have not seen any evidence for any NP effects, which means the masses of the new particles are quite large. The better we can measure these effects, however, the larger the range of masses we can probe. We also look for dark matter both in direct and indirect production.
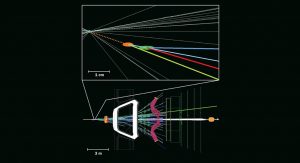
Experimental particle physics at Syracuse University
The experimental particle physics group at Syracuse University started in the mid 1960’s. Experiments were done at Brookhaven National Laboratory, CERN, and Cornell’s CESR facility. In 2004, the group joined the LHCb experiment6 in order to continue our research in the decays of b and c quarks, since opportunities to do so at Fermilab were ended. The LHCb detector is positioned at one of the four places in the LHC where protons collide. An interaction image from a proton-proton collision producing a particle containing a b-quark that decays into a particle containing a c-quark plus one other charged track is shown in Fig. 3.
Our group consists of five faculty members, one research faculty, two postdoctoral fellows, and ten graduate students. The faculty members teach classes for undergraduate and graduate students. Our group has two general research activities: data analysis, and detector R&D and construction. We examine the data to investigate many topics. Then we write papers to share our results with the community. These papers address various topics including CP violation, mention above.
One startling result was the discovery of objects like the proton which could have five quarks rather than three. These are called ‘pentaquarks’. In addition, we have identified objects which are likely to have two quarks and two anti-quarks, called ‘tetraquarks’. These observations contribute to the understanding of theories of strong interactions.7
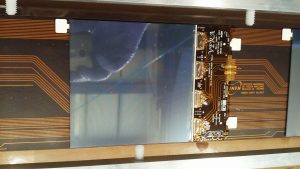
Improvements to detector technology can make dramatic changes in our ability to discover new phenomena. At the same time as we work on data, we also do research and development on new possible adaptations. And when we find good ways of making advances, we request funds to construct new detector elements. Currently, LHCb is upgrading the entire detector in order to be able to take data at 5-10 times the current rate. Our group is leading the effort on the charged particle tracking system in front of the magnet. We detect the tracks with silicon that is 320 microns thick and 10cm x 10cm in transverse dimensions. The silicon has strips that are 190 microns wide, with each one connected to a separate electronics channel. Thus, if a particle goes through it, we can localise its position. The silicon is glued to a support structure called a stave with 14 pieces of silicon on each of the 68 staves (half on the front and half on the back), providing full coverage. One silicon detector with its electronics glued to a stave is shown in Fig. 4. There are approximately ten other projects in the LHCb Upgrade.
This is an interesting time for us. The experiment has found tantalising hints that e−, µ−, and τ− leptons behave differently in certain situations. This violates the Standard Model expectation and would be the first evidence for NP. However, the data are not yet statistically compelling.8 We had hoped to start data taking in 2021, after we finished constructing and installing the new detectors for the upgrade. However, this has been held up because of COVID-19 as CERN is has limited access as all the Universities in LHCb. When we take new data, we should quickly be able to see if these hints go away or a great discovery awaits.
Working in experimental particle physics is a very exciting and rewarding. CERN and LHCb have been most welcoming. We hope to soon get over the current crises, take more data, and make some critical discoveries about the most fundamental states and forces in the Universe.
I thank the US National Science foundation and Syracuse University for support, and my LHCb colleagues for pleasant and helpful collaboration.
References
- Speculative theories of ‘extra dimensions’, where space-time has one or more curled up dimensions added, have mechanisms where particles get different masses. See S. King, ‘Who ordered that?’, CERN Courier (2020), https://cerncourier.com/a/who-ordered-all-of-that
- Howell, ‘What is the big bang theory’, Space.com (2017), https://cerncourier.com/a/who-ordered-all-of-that
- Gnida and K. Jepsen, ‘Charge-parity violation’, Symmetry (2015), https://www.symmetrymagazine.org/article/charge-parity-violation
- Baudis, ‘The Search for Dark Matter’, arXiv:1801.08128
- Other experiments such as ATLAS and CMS do this physics, but mostly on only a few topics rather than LHCb’s broad scope in this area. Another partially competitive experiment is BELLE II in Japan
- https://lhcb-public.web.cern.ch
- Charley, ‘LHC physicists discover five-quark particle, Symmetry (2015), https://www.symmetrymagazine.org/article/july-2015/ lhc-physicists-discover-five-quark-particle
- Bifani, C. Bozzi, G. Ciezarek, and P. Owen, ‘Beauty quarks test lepton universality’, CERN Courier (2018), https://cerncourier.com/a/ beauty-quarks-test-lepton-universality/
Professor Sheldon Stone
Physics Department
Syracuse University
+1 315 443 5972
slstone@syr.edu
Tweet @SyracuseU
https://thecollege.syr.edu/people/faculty/stone-sheldon/
Please note, this article will also appear in the second edition of our new quarterly publication.