In the battle against controlling chemical reactions, researchers have reported, for the first time, the manipulation of the formation rate of urethane molecules in a solution contained inside an infrared cavity.
Controlling chemical reactions to generate new products is currently one of chemistry’s largest challenges.
Developments in this area impact numerous industries. For example, improving the production of catalysts to accelerate chemical reactions and reducing the waste generated in the manufacture of construction materials.
Manipulating the chemical reactivity of molecules
Because of this, different laboratories specialising in the field of polariton chemistry have developed experiments in optical cavities over the last ten years. These experiments aim to manipulate the chemical reactivity of molecules at room temperature, using electromagnetic fields.
Some teams have succeeded in modifying chemical reaction products in organic compounds.
However, no research team has been able to come up with a general physical mechanism to describe the phenomenon and to reproduce it to obtain the same consistent results.
Now, researchers from the University of Santiago, led by principal investigator Felipe Herrera, and the laboratory of the chemistry division of the US Naval Research Laboratory have reported the manipulation of the formation rate of urethane molecules in a solution contained inside an infrared cavity for the very first time.
The discovery proves that selectively controlling the reactivity of certain bonds in a chemical reaction at room temperature in a liquid solvent is theoretically and experimentally possible. This is through the influence of the electromagnetic field vacuum in a narrow range of infrared frequencies.
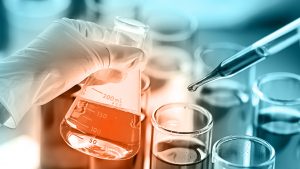
“This theoretical discovery improves our fundamental understanding of the phenomenon over other models that merely explain partial aspects of the experimental observations or simply refute the experimental evidence entirely,” said Herrera.
The work, ‘Modification of ground-state chemical reactivity via light–matter coherence in infrared cavities,’ is published in the journal Science.
Why is controlling chemical reactions a challenge?
When chemical reactions occur, the bonds that connect the atoms in a molecule break and rearrange, forming new substances known as products. Energy is often needed for this process to occur.
Several physiochemical principles have helped us understand how this energy transfer occurs.
There are also principles of reactivity based on the structures of molecules. For example, those proposed by Eyring, Evans, and Polanyi in 1935 are still widely used in all areas of chemistry.
These principles indicate that each reaction between two molecules is independent of the other chemical reactions that occur in a solution.
“That is very valid in almost all situations studied in 80 years and more, but the electromagnetic vacuum creates correlations between the different chemical reactions that happen within the volume of the cavity, and those correlations created by the electromagnetic field, in principle make the traditional assumptions of chemical reactivity questionable,” explained Herrera.
The electromagnetic vacuum creates correlations between the different chemical reactions that happen within the volume of the cavity
“The experimental contribution of this study is the confirmation of the modification of the reaction rates through the interaction with the vacuum of the electromagnetic field confined inside the cavity, using a well-studied chemical reaction, and with more significant changes than those found with other types of reactions,” added Johan Triana, a postdoctoral fellow at MIRO and the University of Santiago.
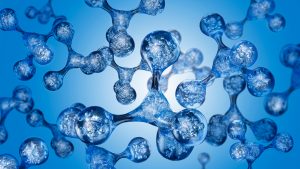
He argued that, in theory, controlling chemical reactions are possible by modifying the dynamics of the bonds that primarily participate in the reaction.
“In the theoretical part, the contribution is the fact that by modifying the dynamics of the chemical bonds that mainly participate in the reaction, through the infrared vacuum, it is possible to control the products.”
Investigating numerous and understanding quantum optics
The first experiments started in 2020. In 2021, new samples were then prepared by Simpkins to ensure that measurements were reproducible and improved the liquid cells where chemical reactions occur.
Herrera and Simpkins then began to have meetings to investigate possible theoretical answers to support the results.
“We decided to start from scratch and build a theory that takes all the physical aspects of quantum optics into consideration, but that under specific conditions reduces to the standard reactivity theory of theoretical chemistry,” explained Herrera.
The work opens new possibilities and more challenges in controlling chemical reactions
Herrera explored the opportunities and challenges that the work will bring about, stating: “We need to develop a sufficiently simple and general theoretical and mathematical framework that any researcher in the world can use to interpret their experiments and hopefully design new types of measurements that no one has yet visualised.
“It would be nice to build a consistent theory that unifies two of the most successful disciplines in modern science: chemical kinetics and quantum physics.”