Researchers are preparing to search for extra-terrestrial planets with the aid of a groundbreaking international astronomical observatory in Turkey.
In 2022, aided by adaptive optics, East Anatolia Observatory (DAG – Doğu Anadolu Gözlemevi) will open its sharp eye to the stars for the first time, to look for extra-terrestrial planets. Here, Professor Laurent Jolissaint, from the University of Applied Sciences Western Switzerland (HES-SO), explains more.
Over the last centuries, astronomical science has been developed mostly in Europe, Russia, and North America, thanks to governments’ support for developing fundamental research, and attracting the most talented researchers. Turkey also had and still has excellent scientists and universities, and Turkish astronomers were not absent from the fundamental research landscape over these years.
Until now, however, it was slightly more difficult for them to obtain data for their scientific programme, due to a lack of modern and large telescopes in the country. It is only by joining international research consortia that Turkish astronomers could gain access to observation time on large facilities, like the European Observatory (EO)’s Very Large Telescope (VLT). This made independent research more challenging.
The DAG project – high-tech astrophysics for Turkey
In 2012, a team of astronomers, led by Dr Cahit Yesilyaprak, Professor of Astronomy at Atatürk University (Erzurum, Turkey), convinced the Turkish Government to invest in a new, large, and modern astronomical telescope, fully equipped with the latest technologies. This telescope, the Eastern Anatolia Observatory, known as Doğu Anadolu Gözlemevi (DAG) in Turkish, is four metres in diameter and will be installed on top of the Karakaya Tepeleri summit (3,170m above sea level) – a mountain near the university city of Erzurum.
The Atatürk University Astronomical Research and Application Center (ATASAM) was created to manage the project, with Dr Yesilyaprak as the Director. Acquisition of high-quality astronomical data, with DAG, will therefore soon be a reality for Turkish astronomers, accessible directly from their home country. Moreover, as we will see, DAG will also have something interesting to share with the international community of astronomers.
Our optical research laboratory at the School of Business and Engineering of the University of Applied Science in Switzerland (HEIG-VD, HES-SO) was contacted by the DAG project management office (Dr Onur Keskin from ISIK University in Istanbul and Dr Cahit Yesilyaprak) at the very start of the project to help in the development of the observatory.
The telescope structure had to be chosen, and it was clear that an adaptive optics system had to be installed to benefit from the maximum optical resolution of the telescope: turbulence in the atmosphere blurs the images of the sky, but adaptive optics is a technique allowing to compensate for these aberrations in real time. Dr Keskin successfully requested a grant for the development of an adaptive optics (AO) system for DAG, and we soon started this AO project together, in parallel with the telescope development.
Faced with the fairly rare chance to develop a new large telescope project, and its AO system at the same time, we immediately decided to push the limits of telescope and astronomical instrument development, slightly beyond the currently standards, without going too far and risking a failed project. DAG clearly should be a 21st century observatory and we made carefully considered choices for the telescope and its AO system.
To start with, as most people know, our planet rotates, and to keep track of the science target during the exposure, the telescope must compensate for this rotation. This is, in principle, a simple thing to do, but, as the telescope rotates, the gravity pulls orientation changes and the telescope structure, mechanics and optics are deformed differently depending on the pointing direction. This has an impact on the pointing accuracy of the telescope, but telescope manufacturers (AMOS in Belgium and EIE in Italy were the two companies selected to build DAG) know how to handle this, and design structures that are stiff enough not to deform beyond some limit. However, this limit is quite extreme: DAG weighs about 40 tons, and the shift of the telescope focal plane should not be larger than a few micrometres, as it would blur the AO corrected star image. Moreover, the primary mirror has to be supported on a set of 78 mechanical push-pull actuators, activated in order to keep the mirror at its perfect shape, whatever the pointing direction – this is called active optics. DAG is therefore an active optics telescope.
There is also the problem of the deformation of the science instruments themselves. If the instruments have to rotate with the telescope, the instrument support structure has to be stiff enough so that the internal optics stay aligned, otherwise the star image would deform and move on the detector during the exposure. The catch, however, is that the stiffer the structure, the more it propagates vibrations – the number one killer of high angular resolution. Our experience told us that the only telescopes without vibrations are dead telescopes. Factors such as driving motors, wind shake, cryocooler pumps of the science instruments, and power transformators all generate mechanical vibrations. So, it would be unreasonable to assume that there are no vibrations of the of the telescope structure.
We therefore decided to mechanically separate, where possible, the instruments from the telescope and locate the instruments on a platform by the telescope side: the so-called Nasmyth platform. This platform is attached to the base mount of the telescope. The instrument cannot be completely separated from the telescope, but the horizontal nature and location of the Nasmyth platform mean the instruments do not move at all. The instrument’s components can be simply fixed on an optical table, as in any optical laboratory.
Fig. 1 shows the telescope structure in the manufacturer premises in Italy, and the Nasmyth platforms can be seen on both sides of the telescope mount. The active optics actuators can also be seen on the back of the telescope. Leaving the telescope through the hollow horizontal axis that can be seen on the picture, the optical beam. While perfectly horizontal, it is still the subject of a rotation around its axis: as seen from the Nasmyth platform, the sky image rotates while the telescope tracks the stars. To compensate for this rotation, we have decided to locate inside the central hole of the horizontal axis itself a so-called optical derotator – an interesting three-mirror system that, when rotated around its axis, rotates the optical beam going through. With the derotator in place, DAG can now be considered as a machine to catch the star light, and prepare it for the instruments, located on a safe and stable environment.
The interest of moderate sized ground-based telescopes in the era of giants and space telescopes
The launch of the James Webb Space Telescope (JWST) is currently taking centre stage in the media, and for good reason. By 2025, it will be followed by the European Extremely Large Telescope (ELT), which will be 40m in diameter and is currently being built by European Southern Observatory (ESO) in the Chilean desert. Together, JWST and ELT will advance our understanding of the universe by a huge step, in every aspect. The reader may therefore wonder why we are constructing small sized ground-based telescopes. There are many very good reasons.
Firstly, if you imagine you are a field biologist, you would not use a microscope to study, for example, the behaviour of wolves, because observing the individual cells of a wolf would not help in the understanding of their society. A good camera would be more suitable. It is the same with astronomical observations: understanding, for instance, galaxies’ evolution does not require an extraordinary angular resolution, simply because there are already many galaxies close enough to be studied in detail with moderate sized telescopes. There is no need for a giant or space telescope. So, the reader may instead question why giant telescopes are being built. The giants and the JWST will have the sensitivity to look at very far away structures, at the beginning of time – a universe that is truly mysterious to us. The tool has to match the task.
The second reason is telescope observation time access. There are many astronomers in the world, with about 100 just in Turkey, but not many large telescopes. Therefore, the competition to get observation time on the space and large telescopes is enormous. So, if a given science programme does not critically need extreme performance, it has to be done on a smaller instrument, increasing the chance to get data, and incidentally relieve the pressure on the behemoths.
Thirdly, there are surveys. It might not be obvious, but some objects in the Universe evolve fast, in all time scales from microseconds to years. There is much to learn by following this evolution. Again, it is not possible to let the JWST or the ELT point on a single object for consecutive days, just to check if something happens – these instruments are in too high demand. This, however, is possible for a smaller telescope. Many medium sized telescopes are dedicated to a certain type of observation only. For instance, in exoplanet research, where data acquisition requires hour long exposures on a single target. There is also statistical surveys. Using the field biologist example again, if you are interested in some tree species, you do not need to sit by the tree and wait for it to grow. You can walk in the forest and examine the same species at different stages of growth and draw your conclusions within a few days. This is the same for astronomical objects. Most of them come into classes. There are rules following the laws of physics, and, by looking at a large enough sample of stars, for instance, conclusions can be drawn. There is not enough telescope time available on the giants for this type of research.
Lastly, there is research and development for new instruments. When an astronomer has an idea for a new instrument, the first tests are done in the laboratory, on an experiment that mimics as much as possible the sky observation conditions. Then the real instrument can be built and installed on a telescope. Most of the time, adjustments are needed at this step – called commissioning – and, if the instrument is aimed at a large telescope, and if the instrument concept is really new, it is highly recommended to carry out the sky validation on a test telescope.
Therefore, as we understand now, size is not all. Telescopes like DAG have a very important role to play in the field of astronomical research – missions that cannot be fulfilled by larger or space telescopes. Astronomers do need them.
First missions for DAG
For the first generation of instruments, DAG is spoilt for choice. As it was decided to equip the telescope with a high order AO system, able to almost completely remove the effect of atmospheric turbulence – in the near infrared wavelengths – it was clear that exoplanet research, as well as the stellar environment exploration, is a good starting point for DAG’s scientific adventure. Exoplanets and star surroundings research requires the highest possible angular resolution. This is just one of many topics and Turkish astronomers, with the help and collaboration of their international colleagues, will decide what to do for the next generation of instruments. There are two scientific programmes that ATASAM is now preparing for DAG: the flexible AO system concept, TROIA, and the stellar coronagraph, PLACID.
TuRkish adaptive Optics for Infrared Astronomy (TROIA) is developed for DAG by OPAM, ISIK University Optomechatronics laboratory, and our optical laboratory at HEIG-VD. An AO system has its limits – in particular, the need for a bright guide star in the sky to serve as a reference to measure the optical aberrations generated by the atmosphere. Turbulence evolves fast, on a timescale of a few milliseconds. Therefore, the measurement has to be done quickly, and the correction command sent to the aberration corrector – a deformable mirror – without losing time. This has to be redone the following millisecond, without interruption. Now, to get a measurement of good quality, there must be enough light received during the acquisition, hence the need for a bright star. Unfortunately, when pointing to any direction in the sky, the chance to find a bright enough star near the astronomical object of interest is low: the sky coverage, as it is called, is only a few percent. There are ways to compensate for this, essentially using several guide stars, and artificial stars. But these techniques are quite complex, and, for a new observatory, it is best to first master the simplest system.
We decided that, if we were going in this direction, we should choose the best possible option in terms of technology, which is why we selected a new kind of aberration sensor – the so-called pyramid wavefront sensor (P-WFS), and a high-order, high-amplitude deformable mirror, based on a magnetic actuators technology exclusively developed by the company ALPAO, in France.
The unique feature of the P-WFS is that its range of sensitivity can be adapted to the amplitude of the aberration. For example, if you want to measure the height of your children, you need a decimetre rule, but if you want to measure the diameter of a pin, you would need something with a low range but high resolution. The P-WFS can do both, while other sensors cannot, so you always get the highest possible accuracy, regardless of the aberration amplitude. Our DM has 468 actuators and can deform its surface by an amplitude of ten micrometres, which means that, if the turbulence is strong, it can be corrected and if the measurement from the P-WFS is good enough, fine details of the wavefront can be compensated as well, washing almost completely the turbulence. Last but not least, we aim at training a neural network – using Machine Learning – such that the system itself decides on the P-WFS range and the number of DM modes to correct, based on the guide star brightness, wind velocity, and strength of the turbulence. This automated AO system would be a premier not only for DAG, but for AO research in general.
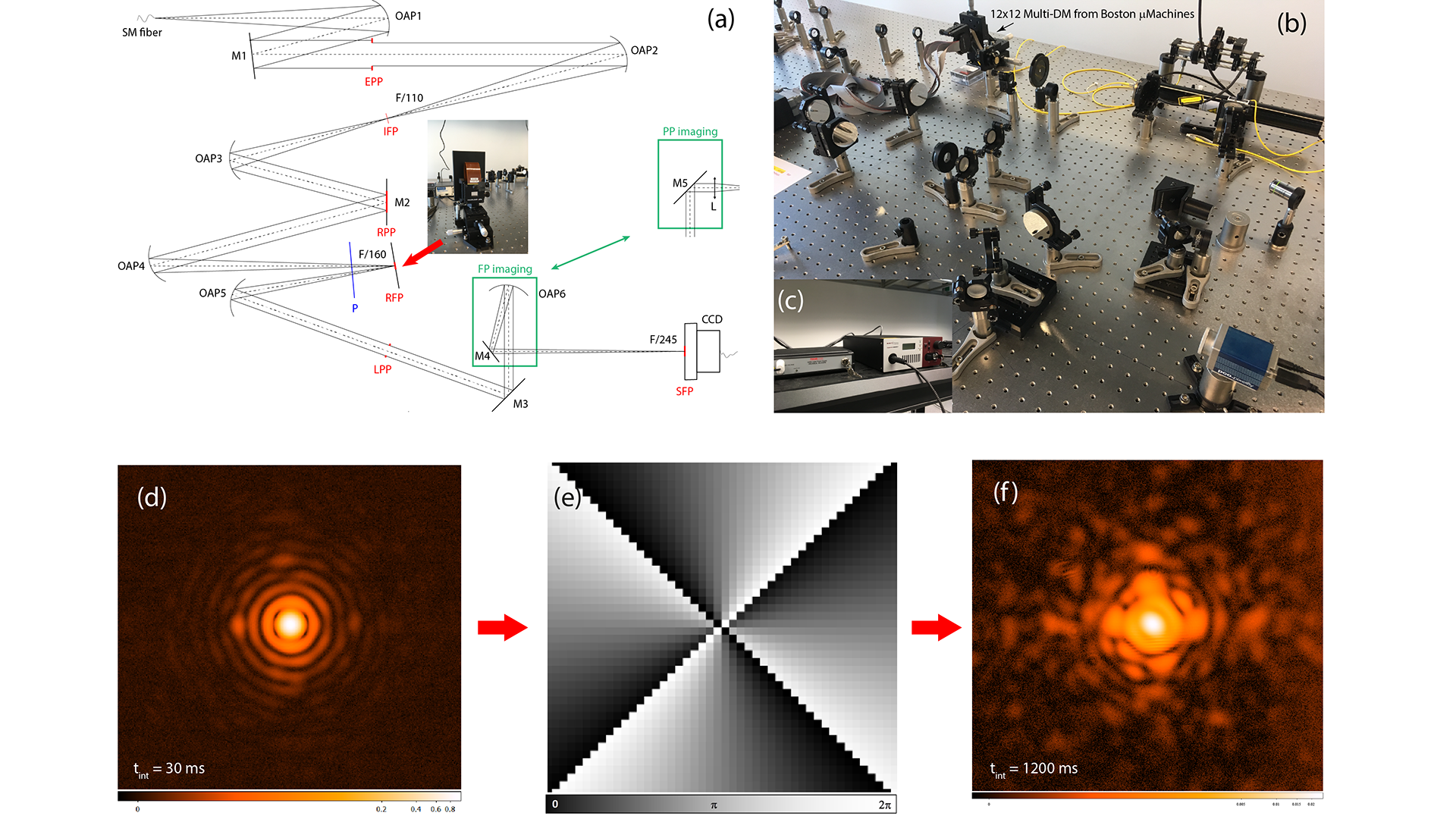
Now that DAG is equipped with a high order AO system, it is possible to carry out direct exoplanet detection – by imaging. We will not see any details on the exoplanets – even the 40m ELT will not have enough angular resolution for this – but we will have enough resolution to separate in the image the planet from its host star. The problem is that stars are so much brighter than their planets – on the order of a million or a billion, the star light has to be hidden if one wants to see the light emitted by the planet itself. This is called coronagraphy – a technique pioneered by the French astronomer Bernard Lyot back in the 1930s to hide the Sun image in his instrument, so the faint Sun’s corona could be seen without the telescope camera picture being completely blinded. This technique has been adapted by astronomers for exoplanet research since the 1990s and has allowed for the first detection of circumstellar discs of dust around nearby stars, where planets form by accretion.
PLACID is the name of the coronagraph that we will put after DAG AO. Here, we are again using this opportunity to test a novel coronagraph technique developed by Dr Jonas Kühn, from the University of Bern, with whom we are teaming at HEIG-VD. The idea is that the star light in a coronagraph is hidden by a mask that blocks the light or makes the light beam interfere with itself, making light disappear – a bit similarly to the antireflection coating one has on our glasses. Dr Kühn proposes to use, instead of a fixed mask, a liquid crystal array where the structure of the coronagraph mask can be changed at will, simply by programming the array, like on a computer liquid crystal screen.
Looking at Fig. 2, what is the advantage? Firstly, different types of masks are of interest depending on the type of star system we want to look at, and there is no longer a need to replace the masks in the instrument. Secondly, a fascinating note is that using a mask to hide the light of the two components of a binary star can be programmed, meaning that it becomes possible to study planets in multiple star systems – let’s think about the famous Tatoïne in the Star Wars saga. This is not possible or very difficult with physical masks, which have to be machined. Knowing that two out of three of the stars of our Galaxy are in couple with other stars, this technique offers very interesting perspectives.
To finish, as one may expect, DAG scientists are very excited by the perspective that the new observatory will offer. It will serve as a top-class observatory for fundamental research in astrophysics and instrumentation development. First light of the telescope is planned for the end of 2022, and 2023 will be the year of the sky commissioning of TROIA and PLACID. Let the adventure begin!