The Barta group outlines novel lignocellulose valorisation concepts, innovative synthesis pathways and fundamentally new zero-waste functionalisation methods towards circular bio-products, developed within their research group for a sustainable chemical industry.
Achieving energy and resource security, and mitigating climate change and environmental pollution are grand societal challenges now and for decades to come. Fundamental research in chemistry and chemical engineering will play a vital role in addressing these key challenges. The chemical industry will be at the centre of this transformation and urgently needs to abandon its linear, fossil-fuel-based enterprise and gear towards utilising renewable feedstocks efficiently, and simultaneously implement sustainability and green chemistry in their future endeavours. In the long term, this adaptation will bring along economic benefits, by reducing import dependencies, creating new employment in related sectors, and increasing waste utilisation and upcycling strategies, ultimately leading to circular economy strategies.1,2
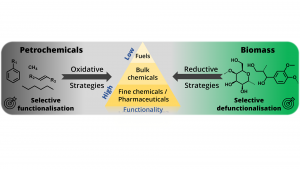
Addressing the chemical industry’s transformation on the fundamental scientific level is far from trivial. Traditional petrochemical processes are designed to add functionality to simple and well-defined, crude oil-derived hydrocarbons, typically employing oxidative routes.3 In stark contrast, renewable resources, such as lignocellulose, are inherently more functionalised (i.e. oxygenated) and occur as robust and structurally complex biopolymers, which require distinctly different processing/biorefining strategies.4 Thus, fundamental research needs to address the development of efficient fractionation and depolymerisation methods (requiring cleavage of specific bonds) and selective deoxygenation/defunctionalisation strategies.3 Undoubtedly, the inherent structural characteristics of renewables must be effectively exploited to add functionality directly, in an atom-economic and ‘waste-minimised’ manner. This is an opportunity to rapidly connect to higher-value products by sustainable pathways whilst minimising the formation of harmful side-products.3–5
Lignocellulose: A challenge and opportunity
Nature itself provides us with the impactful opportunity to mitigate climate change and its effects by carbon capture through photosynthesis. It was recently postulated that trees have a carbon capture potential of 200 billion tons at plant maturity, assuming a global canopy-based tree cover with a potential of 4.4 billion hectares and an estimated ten tons average annual potential of dry biomass production per hectare.6,7 Dry biomass being essentially made out of cellulose, hemicellulose and lignin does not compete with the food supply, and thus is an ideal target for the production of sustainable bio-based chemicals, fuels and materials.8 However, the currently available biorefinery approaches are still deemed unattractive in the chemical industry, due to the inefficient processing/conversion routes compared to the traditional petrochemical refinery.6
When constructing novel biorefinery schemes, the sustainability of the overall process should be critically assessed. There should be novel ways to meaningfully connect to existing bulk chemicals and products. At the same time, the inherent structural complexity of biomass should allow the development of ‘emerging products’ with a significantly improved environmental footprint, and improved chemical and biological degradability, taking into account the improvement of health and safety aspects of the processes and products. Only by increasing overall sustainability and efficiency, both in fractionation and downstream processing, combined with expedient product properties, will it be possible to reach the economic feasibility that is necessary to compete with established petrochemical refinery schemes.
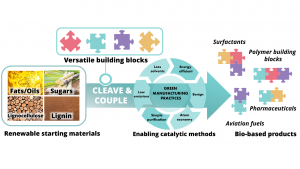
Our research aims
Fundamental and high-level applied research in our group focuses on the development of sustainable biorefinery schemes based on lignocellulosic biomass, by focusing on key challenges in feedstock fractionation, depolymerisation, and sustainable downstream conversion routes.8–10
Our methods aim to maximise renewable carbon utilisation by achieving high selectivity to well-defined platform chemicals, that maintain to a certain extent, the inherent structural features of biomass and serve as a handle for subsequent catalytic conversion routes that connect to products for diverse applications (aviation fuels, polymer building blocks, pharmaceutical intermediates).8,11 This strategy, aligned with the 12 principles of green chemistry, ensures the reduction of unwanted side products and increases overall sustainability in the chemical industry.
ERC Starting Grant CatASus – Cleave & Couple
Amines are omnipresent in the chemical industry, widely used in agrochemicals, pharmaceuticals, polymers, and surfactants. Yet, surprisingly, at the start of our ERC Starting Grant, 2015 (CatASus), there was a lack of catalytic strategies to obtain these compounds from lignocellulosic biomass. Lignocellulosic biomass is highly oxygenated and provides an excellent resource for the manufacture of amines. We have recognised that, by developing mild depolymerisation strategies to platform chemicals rich in aromatic and aliphatic alcohols, these intermediates could be directly coupled with ammonia to result in a range of valuable amines.
Thus, our project focused to address these two key challenges, dubbed ‘CLEAVE and COUPLE’, where ‘CLEAVE’ refers to the development of novel mild depolymerisation strategies, whilst ‘COUPLE’ entails the development of atom-economic methodologies to couple the biomass-derived alcohols with ammonia or other coupling partners, to access value-added products.4 Importantly, in both stages, homogeneous and heterogeneous catalyst development focused on the use of earth-abundant metals, as the only sustainable option in light of the expected noble metal scarcity in the coming decades.
The project brought significant benefits in the field of lignin valorisation by introducing new lignin-derived platform chemicals, and increasing selectivity and yield to monoaromatic compounds, while suppressing unwanted recondensation phenomena by stabilisation of reactive intermediates during depolymerisation.12–15 For example, by trapping reactive phenolic C2-aldehydes formed during acidolysis, in the form of their cyclic acetals using diols or polyols, it was possible to give access to well-defined mono-aromatic compounds, which are amenable to further functionalisation. Another example is the use of copper-doped porous metal oxides, derived from synthetic hydrotalcites, to enhance lignin depolymerisation or reductive catalytic fractionation to aromatic and aliphatic alcohols and subsequent catalytic strategies to access aviation fuels, and valuable aromatic monomers, with a focus on amines.8 The synthesis of valuable amines derived from lignin was further expanded upon in multiple studies involving Raney® Nickel, targeting cyclohexylamine,16 1,4-cyclohexanediamine,17 dihydroconiferyl amine,18 as well as various other diamines.19
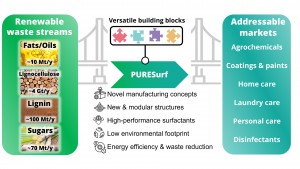
Furthermore, the use of lignin-derived chemicals to produce polymers was investigated.9,10 Fully bio-based polybenzoxazines were produced from 4,4´-methylenebiscyclohexanamine (MBCA) that exhibited comparable properties compared to commercial analogues. MBCA was derived in a two-step process via catalytic funnelling of lignin-derived bisphenols into 4,4´-methylenebiscyclohexanol followed by selective amination with ammonia.9 In a related study, bio-based PET analogues were targeted that displayed promising chemical recyclability in methanol. A noble metal-free catalytic sequence was employed to obtain a simple diol (4-(3-hydroxypropyl) cyclohexan-1-ol) from lignocellulose that was co-polymerised in the last step with potentially bio-derived diesters (terephthalic acid- and furan dicarboxylic methyl ester).10
ERC PoC PURE
Building on the results acquired during the ‘Cleave and Couple’ programme, we next set out to prototype zero-waste pathways to valuable products with high potential in the chemical industry.
Surfactants are an essential cog in the wheel of modern society, with an annual production volume of about 40 million tons, widely utilised in applications such as home, laundry, personal care, agrochemicals, paints, dyes, and pharmaceuticals. Currently, industrially applied surfactants have been optimised for high performance, which unfortunately neglects the concepts of sustainability, resulting in suffering from polluting manufacturing routes and raw material supply problems.20
Thus, the ERC proof-of-concept project, named PURE, aimed to address the development of novel, sustainable pathways to produce high-performance, 100% surfactants which can be derived from locally available renewable raw material waste streams generated in large quantities, such as waste cooking oil, lignocellulose, or lignin side-products of papermaking.
Within the project, a library of 40 surfactants, including non-ionic (amine-oxides), zwitterionic (amino-acid based) and anionic (sulfonates) compounds, was generated by innovative and sustainable pathways, leading to two PCT (PCT/EP2022/076255, PCT/EP2022/076252) and two Austrian PRIO patent applications. The performance of the novel bio-based surfactant structures was evaluated in terms of solubility, cold/hot temperature behaviour (Krafft & cloud point), hard water tolerance, viscosity, surface- and interfacial tension, as well as critical micelle concentration (CMC). Initial results have shown excellent low-temperature behaviour and CMCs up to two orders of magnitude lower than petrochemical representatives, with some examples displaying a gel-like state already at 1wt% concentration. Beyond that, also the feasibility, scalability, and commercial potential of this approach were examined. A series of interviews have demonstrated the apparent need for novel bio-surfactants in the chemical industry, with sustainability being a major market driver, especially in Europe.
EIC Transition Grant PureSurf
This promising traction inspired us to further pursue our endeavours, to impact the surfactant market sustainably, with the credo ‘superior performance and clean conscience’.
This culminated in the prestigious European Innovation Council’s Transition Grant in 2021 (grant agreement no.: 101058142).
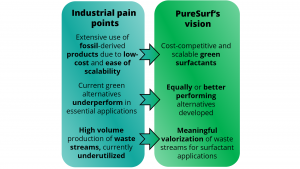
The aim of this project is to produce industrially attractive tailormade bio-based surfactants, based on the concepts developed and insights gained within CatASus and PURE. The key challenges of developing such green and sustainable products for the chemical industry are the competitive costs of petrochemicals, fluctuating or limited feedstock availability, process scalability, and product purity. Thus, this ambitious goal is only achievable if the envisioned manufacturing processes are feedstock flexible, both design philosophy and synthetic strategy are rooted in the 12 principles of green chemistry and take feedstock availability into account.
Furthermore, close co-operation with industrial partners and/or customers is considered essential for success at this stage because external feedback early in the development cycle ensures market relevance of the final product. Therefore, reaching the kg-scale for the developed synthesis routes, the evaluation of the obtained products in industrial applications, and rigorous analysis of their performance, as compared to already existing fossil-based or bio-based surfactants, is considered a top priority of the project. Additionally, we aim to investigate the techno-economic feasibility and scalability of the synthesis routes, with projections towards large-scale manufacturing. Similarly, great attention is devoted to understanding the physicochemical properties of the new structures, as well as their toxicity, biodegradability and the overall environmental footprint of the developed methods.
Our ultimate goal is to provide novel, circular and bio-based strategies for the chemical industry, undoubtedly attractive for industrial partners, which strive to move away from fossil-based surfactants towards cleaner, greener, more sustainable solutions whilst maintaining the performance of their manufactured products.
Parties interested in our methodology or testing our prototypes in formulations, or supporting our work, are encouraged to get in contact with us via e-mail at office@puresurf.eu.
Research within the Barta Group has received funding from the European Union‘s H2020 research and Innovation program under grant agreement no. 67883 and 875649 as well as European Union’s Horizon Europe research and Innovation program under grant agreement no. 101058142.
References
1: Ellen McArthur Foundation. The New Plastics Economy: Rethinking the Future of Plastics and Catalysing Action.
2: The European Green Deal; [Publications Office of the European Union], 2020.
3: Barta, K.; Ford, P. C. Catalytic conversion of nonfood woody biomass solids to organic liquids. Accounts of chemical research 2014, 47 (5), 1503–1512. DOI: 10.1021/ar4002894.
4: Sun, Z.; Barta, K. Cleave and couple: Toward fully sustainable catalytic conversion of lignocellulose to value added building blocks and fuels. Chemical communications 2018, 54 (56), 7725–7745. DOI: 10.1039/C8CC02937G.
5: Sun, Z.; Fridrich, B.; Santi, A. de; Elangovan, S.; Barta, K. Bright Side of Lignin Depolymerization: Toward New Platform Chemicals. Chemical reviews 2018, 118 (2), 614–678. DOI: 10.1021/acs.chemrev.7b00588.
6: Van Meerbeek, K.; Muys, B.; Hermy, M. Lignocellulosic biomass for bioenergy beyond intensive cropland and forests. Renewable and Sustainable Energy Reviews 2019, 102, 139–149. DOI: 10.1016/j.rser.2018.12.009.
7: Bastin, J.-F.; Finegold, Y.; Garcia, C.; Mollicone, D.; Rezende, M.; Routh, D.; Zohner, C. M.; Crowther, T. W. The global tree restoration potential. Science 2019, 365 (6448), 76–79. DOI: 10.1126/science.aax0848.
8: Sun, Z.; Bottari, G.; Afanasenko, A.; Stuart, M. C. A.; Deuss, P. J.; Fridrich, B.; Barta, K. Complete lignocellulose conversion with integrated catalyst recycling yielding valuable aromatics and fuels. Nature Catalysis 2018, 1 (1), 82–92. DOI: 10.1038/s41929-017-0007-z.
9: Wu, X.; Galkin, M. V.; Barta, K. A well-defined diamine from lignin depolymerisation mixtures for constructing bio-based polybenzoxazines. Green Chemistry Catalysis 2021, 1 (7), 1466–1479. DOI: 10.1016/j.checat.2021.10.022.
10: Wu, X.; Galkin, M. V.; Stern, T.; Sun, Z.; Barta, K. Fully lignocellulose-based PET analogues for the circular economy. Nature communications 2022, 13 (1), 3376. DOI: 10.1038/s41467-022-30735-4.
11: Elangovan, S.; Afanasenko, A.; Haupenthal, J.; Sun, Z.; Liu, Y.; Hirsch, A. K. H.; Barta, K. From Wood to Tetrahydro-2-benzazepines in Three Waste-Free Steps: Modular Synthesis of Biologically Active Lignin-Derived Scaffolds. ACS central science 2019, 5 (10), 1707–1716. DOI: 10.1021/acscentsci.9b00781.
12: Santi, A. de; Galkin, M. V.; Lahive, C. W.; Deuss, P. J.; Barta, K. Lignin-First Fractionation of Softwood Lignocellulose Using a Mild Dimethyl Carbonate and Ethylene Glycol Organosolv Process. ChemSusChem 2020, 13 (17), 4468–4477. DOI: 10.1002/cssc.201903526.
13: Lahive, C. W.; Deuss, P. J.; Lancefield, C. S.; Sun, Z.; Cordes, D. B.; Young, C. M.; Tran, F.; Slawin, A. M. Z.; Vries, J. G. de; Kamer, P. C. J.; Westwood, N. J.; Barta, K. Advanced Model Compounds for Understanding Acid-Catalysed Lignin Depolymerisation: Identification of Renewable Aromatics and a Lignin-Derived Solvent. Journal of the American Chemical Society 2016, 138 (28), 8900–8911. DOI: 10.1021/jacs.6b04144.
14: Deuss, P. J.; Lancefield, C. S.; Narani, A.; Vries, J. G. de; Westwood, N. J.; Barta, K. Phenolic acetals from lignins of varying compositions via iron(iii ) triflate catalysed depolymerisation. Green Chemistry. 2017, 19 (12), 2774–2782. DOI: 10.1039/c7gc00195a.
15: Deuss, P. J.; Scott, M.; Tran, F.; Westwood, N. J.; Vries, J. G. de; Barta, K. Aromatic monomers by in situ conversion of reactive intermediates in the acid-catalysed depolymerisation of lignin. Journal of the American Chemical Society 2015, 137 (23), 7456–7467. DOI: 10.1021/jacs.5b03693.
16: Wu, X.; Bruyn, M. de; Barta, K. One-Pot Catalytic Conversion of Lignin-Derivable Guaiacols and Syringols to Cyclohexylamines. ChemSusChem 2022, 15 (18), e202200914. DOI: 10.1002/cssc.202200914.
17: Wu, X.; Bruyn, M. de; Hulan, J. M.; Brasil, H.; Sun, Z.; Barta, K. High yield production of 1,4-cyclohexanediol and 1,4-cyclohexanediamine from high molecular-weight lignin oil. Green Chemistry. 2023, 25 (1), 211–220. DOI: 10.1039/D2GC03777G.
18: Wu, X.; Bruyn, M. de; Barta, K. Primary amines from lignocellulose by direct amination of alcohol intermediates, catalyzed by RANEY® Ni. Catalysis science & technology 2022, 12 (19), 5908–5916. DOI: 10.1039/d2cy00864e.
19: Wu, X.; Bruyn, M. de; Barta, K. A Diamine-Oriented Biorefinery Concept Using Ammonia and Raney Ni as a Multifaceted Catalyst. Chemie-ingenieur-technik 2022, 94 (11), 1808–1817. DOI: 10.1002/cite.202200091.
20: Arthur D. Little, I.; Soap and Detergent Association. Environmental and Human Safety of Major Surfactants: Anionic surfactants. pt. 1. Linear alkylbenzene sulfonates. pt. 2. Alcohol ethoxy sulfates. pt. 3. Alkyl sulfates; Arthur D. Little, Incorporated, 1991.
Please note, this article will also appear in the thirteenth edition of our quarterly publication.