Quantum mechanics explores the fundamental nature of matter and energy at the smallest scales. Key concepts include quantised particles and probabilistic wave functions, as governed by the Schrödinger equation.
Notable principles of quantum mechanics, such as wave-particle duality and the uncertainty principle, challenge classical physics. Quantum phenomena like tunnelling and entanglement enable groundbreaking technologies, including quantum computing and cryptography.
The origins of quantum theory can be traced back to pivotal contributions by Max Planck, Albert Einstein, and others.
By understanding these critical theories, we can gain insight into the perplexing yet fascinating domain of quantum mechanics and its vast potential in our future.
Quantum physics fundamentals
Quantum physics, the branch of science that investigates the behaviour of matter and energy at the smallest scales, introduces a groundbreaking framework where classical mechanics no longer apply.
At the core of this field lies the concept of quantisation, where particles such as electrons are described by discrete packets referred to as quanta. These quantum objects are governed by probabilistic wave functions and the Schrödinger equation, allowing them to exist in multiple states simultaneously—an idea recognised as superposition.
One of the intriguing phenomena in quantum physics is quantum tunnelling, where particles pass through potential barriers that would be insurmountable in classical physics. This effect is fundamental to technologies like the scanning tunnelling microscope, which allows for imaging surfaces at the atomic level.
Quantum decoherence describes the process by which a quantum system loses its superposition state due to interaction with its environment, shifting it to a classical state. This phenomenon is a significant challenge in the development of quantum computing, where maintaining coherence is essential for performing calculations that far exceed the capabilities of classical computers.
Quantum teleportation, another fascinating concept, involves the transfer of quantum information between particles over distance, leveraging the phenomenon of entanglement. This principle has profound implications for the future of secure communication.
Quantum cryptography capitalises on the principles of quantum mechanics to develop secure communication methods that are theoretically immune to eavesdropping. This field promises to revolutionise data security.
The origins of quantum mechanics
At the dawn of the 20th century, classical physics’ inadequacies in explaining certain phenomena led to the birth of quantum theory. This revolutionary framework emerged from a series of historical breakthroughs and early experiments that challenged the prevailing notions of classical mechanics.
One of the pivotal moments was Max Planck’s introduction of quantised energy levels in 1900, laying the cornerstone for quantum theory. Planck’s work addressed the black-body radiation problem that classical physics could not resolve, marking the first significant conceptual shift towards quantum mechanics.
Albert Einstein further advanced this nascent field in 1905 through his explanation of the photoelectric effect, which demonstrated that light could be understood as discrete packets of energy called quanta or photons. This revelation underscored the inadequacy of the wave theory of light and reinforced the need for a new theoretical framework.
Niels Bohr’s 1913 model of the atom, which introduced quantised orbits for electrons, was another foundational principle that provided deeper insights into atomic structure and stability.
Subsequently, Erwin Schrödinger’s wave function formalism, published in 1926, offered a robust mathematical description of quantum systems and their evolution, leading to significant theoretical advancements.
These collective efforts culminated in the formulation of quantum mechanics, a probabilistic framework that profoundly altered our understanding of particle behaviour and interactions at the microscopic level.
Wave-particle duality
One of the most intriguing aspects of quantum mechanics is wave-particle duality, which posits that particles such as electrons exhibit both wave-like and particle-like properties. This concept challenges the classical physics paradigm by demonstrating that particles can exhibit wave characteristics, such as interference patterns, a phenomenon traditionally attributed solely to waves.
The cornerstone of wave-particle duality is the double-slit experiment, which provides compelling experimental evidence. When electrons are fired through two closely spaced slits, they create an interference pattern on a detection screen, a clear indication of their wave-like behaviour.
Conversely, when observed, these same electrons appear to act as discrete particles, showcasing their dualistic nature.
This dualistic nature underscores the complexity of quantum mechanics and highlights the need to rethink conventional concepts of particles and waves.
Through these insights, wave-particle duality forms a critical pillar in the broader understanding of quantum physics.
Superposition and entanglement
Superposition and entanglement are cornerstones of quantum mechanics. They present phenomena that defy classical intuition and underpin the field’s most revolutionary advancements.
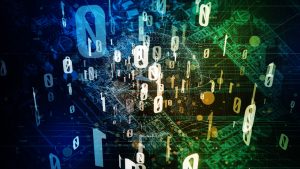
In quantum physics, superposition refers to the ability of quantum particles to exist in multiple states simultaneously. This property is pivotal in quantum computing, where quantum bits, or qubits, harness superposition to perform complex computations in parallel, leading to exponential speedups in solving certain problems compared to classical computers.
Quantum entanglement, another fundamental concept, occurs when particles become interconnected in such a way that the state of one particle instantly influences the state of another, regardless of the distance separating them. This phenomenon, known as quantum entanglement, is instrumental in the development of quantum communication and quantum information processing.
Entangled particles share correlated properties, enabling secure communication methods such as quantum key distribution, which can detect eavesdropping attempts due to the disturbance it would cause in the entangled states.
Quantum algorithms exploit both superposition and entanglement to enhance computational efficiency. For instance, Shor’s algorithm for factoring large numbers and Grover’s algorithm for database searching demonstrate how quantum systems can outperform classical counterparts.
Superposition allows these algorithms to evaluate many possibilities simultaneously while guaranteeing the coherence and interdependence of qubits, maintaining the integrity of quantum information throughout the computation process.
The Schrödinger Equation
The Schrödinger Equation stands as a cornerstone in quantum mechanics, offering a profound mathematical framework for understanding the dynamic behaviour of quantum systems.
Formulated by Erwin Schrödinger in 1925, this pivotal equation encapsulates the essence of quantum evolution, describing how a quantum system evolves over time. It is instrumental in predicting the behaviour of particles at the microscopic level, revealing the intricate dance of wave functions and probability amplitudes.
At the heart of the Schrödinger Equation is the concept of the wave function. This mathematical entity encodes the probability amplitude of finding a particle in a particular state or position. This wave function is central to determining the likelihood of various outcomes in quantum experiments and forms the bedrock of quantum predictions.
The Schrödinger Equation can be expressed in both time-dependent and time-independent forms, each serving distinct purposes in the analysis of quantum systems. The time-dependent Schrödinger Equation describes how the wave function evolves with time, while the time-independent form is often used to solve for the system’s stationary states.
The Uncertainty Principle
The Uncertainty Principle, a cornerstone of quantum mechanics formulated by Werner Heisenberg, delineates the fundamental limitations in simultaneously measuring certain pairs of complementary properties, such as a particle’s position and momentum, with arbitrary precision.
This principle underscores the quantum uncertainty inherent in particle dynamics, challenging the classical physics notion that such properties can be measured with exactitude.
Heisenberg’s principle asserts that the more precisely one knows a particle’s position, the less precisely one can know its momentum, and vice versa. This position momentum dilemma arises from the wave-like nature of quantum objects, where particles are described not by definite positions and velocities but by wave functions that encapsulate probabilities.
Consequently, any attempt to measure one property with high precision inevitably disturbs the other, introducing an intrinsic quantum uncertainty.
At its core, the Uncertainty Principle fundamentally alters our understanding of predictability and measurability at the quantum level. It implies that there is a limit to the precision with which we can simultaneously know a particle’s position and momentum. This limit is not due to experimental imperfections but a fundamental property of nature itself, rooted in the principles of quantum mechanics.
The double-slit experiment
Amid the foundational experiments that reveal the enigmatic nature of quantum mechanics, the double-slit experiment stands as a pivotal demonstration of wave-particle duality.
Originally conceived by Thomas Young in the early 19th century to study light, it has since become a cornerstone in quantum physics, elucidating the dual nature of quantum particles such as electrons and photons.
In the double-slit experiment, particles are emitted towards a barrier with two slits. When both slits are open, and no observation mechanism is in place, the particles create an interference pattern on the detection screen, characteristic of wave behaviour. This pattern is akin to ripples overlapping on a water surface, with alternating bands of high and low intensity, indicating the superposition of quantum states.
However, the quantum mysteries deepen when an observation mechanism is introduced to determine through which slit the particle passes. The act of observing collapses the interference pattern, resulting in two distinct clusters on the screen, indicative of particle behaviour. This phenomenon, known as observation collapse, highlights the profound impact of measurement on quantum systems.
Quantum technologies and applications
Quantum technologies, rooted in the principles of quantum mechanics, are revolutionising various sectors by offering unprecedented computational power, enhanced security, and precision measurement capabilities. These technologies harness quantum phenomena such as superposition and entanglement to develop groundbreaking applications across multiple fields.
Quantum computing advancements are at the forefront of this revolution. Unlike classical computers, which use bits as the smallest unit of data, quantum computers employ qubits. Qubits can exist in multiple states simultaneously, enabling them to solve complex problems at an exponential speed. This dramatic increase in computational power holds the potential for solving currently intractable problems in cryptography, optimisation, and materials science.
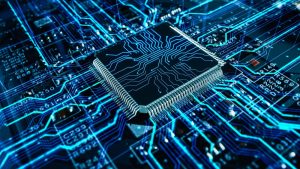
Quantum cryptography developments leverage the principles of quantum mechanics to ensure secure communication. Quantum Key Distribution (QKD) uses entangled particles to detect eavesdropping, making it theoretically impossible for an interceptor to access the communication without being detected. This guarantees unparalleled quantum communication security.
Quantum sensor innovations exploit quantum phenomena to achieve high-precision measurements. These sensors can enhance imaging technologies, improve navigation systems, and enable more accurate detection of environmental changes. The precision offered by quantum sensors is transforming fields such as medicine, where they can be used for early disease detection, and geology, where they can predict seismic activities with greater accuracy.
These quantum technology applications are not confined to theoretical research – they are already impacting practical fields and propelling industries towards unprecedented advancements.
The future of quantum advancements
Quantum physics fundamentally challenges classical conceptions of reality by introducing phenomena such as superposition, wave-particle duality, and probabilistic behaviour.
Governed by the Schrödinger equation and demonstrated through experiments like the double-slit experiment, quantum systems reveal the profound impact of observation on outcomes.
These principles not only illuminate the microscopic domain but also propel advancements in quantum technologies, redefining the understanding of the universe’s underlying principles and opening new frontiers in scientific exploration.