Dr Paschal Coyle from the Centre de Physique des Particules de Marseille introduces the KM3NeT – a large astronomical telescope currently being built under the sea to explore the neutrino universe.
Deep in the abysses of the Mediterranean Sea, scientists across Europe are busy constructing the Kilometre cube Neutrino Telescope (KM3NeT) – one of the largest astronomical telescopes ever built.
Building a telescope so deep in such a hostile environment is not easy. Why have scientists embarked on such a challenging construction? The reason is to catch as many neutrinos as we possibly can.
Neutrinos are a fundamental particle of matter. They have no charge, no size, almost no mass and interact via the weakest of the interactions. Even though neutrinos are the closest thing to nothing we can imagine, they are key to understanding the workings of the Universe. Although we literally swim in an ocean of neutrinos, with billions of them passing through our bodies every second, we know less about these particles than any of the other constituents of matter. For more than 80 years, physicists around the world have been chasing these mysterious and elusive particles. Neutrinos offer us the possibility of exploring the farthest reaches of the Universe, to figure out the origin of cosmic rays, and to probe the heart of the Sun and the inner workings of our own planet. Understanding neutrinos will help us explain how stars explode in supernovae, provide insights into the prevalence of matter over antimatter, and reveal the nature of dark matter.
A European research infrastructure
KM3NeT is a research infrastructure (RI) with two neutrino detectors, ARCA and ORCA. ARCA is located at a depth of 3,500m offshore of Sicily, while ORCA is located at a depth of 2,450m offshore of the South of France. For ARCA, a seawater volume of 1 km3 is sparsely instrumented, which is optimal for the detection of TeV-PeV cosmic neutrinos. In contrast, the much smaller water volume of 7 Mton of ORCA is densely instrumented, which is necessary for the study of sub-TeV neutrinos.
About 300 scientists from 47 universities and 19 countries participate in the KM3NeT Collaboration. Originally European, the Collaboration has enlarged to include institutes from Africa, Australia, China and the USA. KM3NeT was supported by the EU for its design study phase and preparatory phase and is currently supported via an INFRADEV. It was selected as a priority RI on the 2006 and 2016 ESFRI (European Strategy Forum for Research Infrastructures) roadmaps. KM3NeT has also played a leading role in many EU-funded projects. The main countries contributing to the construction cost of the RI are France, Italy, and the Netherlands, with a significant contribution from various regional funds from the host sites in Provence and Sicily. The RI is planning to adopt an AISBL legal entity before the end of 2025.
Innovative technology
The key technology of KM3NeT is its many thousands of optical modules and beach-ball-sized spherical glass spheres, each hosting 31 ultra-sensitive light sensors pointing in different directions. Installed in a giant three-dimensional grid of vertical neutrino detection lines, these ‘eyes’ catch the faint glow of bluish Cherenkov light created when neutrinos interact with the seawater surrounding the telescope (see Fig. 1).
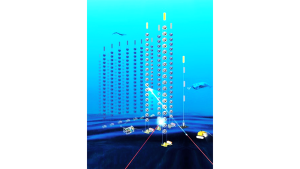
18 optical modules are hosted on each detection line. The lines are anchored to the seafloor and held taut by a submerged buoy. The bases of the lines are connected via cables on the seafloor to junction boxes, from which telecommunication cables, many tens of kilometres long, bring the data to shore along optical fibres. Information on every single photon detected is transmitted to the shore stations, where trigger algorithms are applied to select interesting events for offline analysis.
The final ARCA detector will comprise 230 detection lines spaced about 100m from each other on the seafloor. The ORCA detector has half as many lines, and their spacing is four times smaller. As of October 2024, 33 and 24 lines are deployed in ARCA and ORCA, respectively.
Unlike conventional telescopes KM3NeT does not need to be pointed in a particular direction; it can ‘see’ in all directions at the same time. Nevertheless, due to a large background noise from downgoing cosmic rays, its clearest vision is for upgoing neutrinos that have travelled through the Earth.
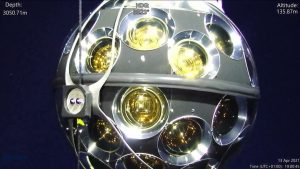
Fundamental neutrino physics
Neutrinos come in three different types or flavours: electron, muon, and tau-neutrinos. About thirty years ago, it was discovered that neutrinos can transform from one flavour to another as they travel. This fascinating behaviour was not at all predicted by the Standard Model of particle physics. We know now that the different neutrino flavours are actually quantum superpositions of three unequal mass states. As the mass states propagate at different speeds, phase differences accumulate between their mass and flavour states, ultimately leading to an effect where the observed neutrino flavour appears to change back and forth over time, referred to as ‘oscillations’. As their experimental signatures are different, KM3NeT can distinguish the flavour of the detected neutrinos.
Atmospheric neutrinos are abundantly produced in the Earth’s atmosphere as decay products of cosmic ray interactions and come in mostly muon and electron flavours. As they pass through the Earth, the relative abundance of the neutrino flavours are modulated through oscillations. For the GeV-scale neutrinos detected by ORCA, the primary effect observed is the oscillation of muon neutrinos into tau neutrinos over distances comparable to the Earth’s diameter. The strength and frequency of this modulation are characterised by the so-called atmospheric oscillation parameters. Already with only its 6-line data, KM3NeT/ORCA has recently published a competitive measurement of these parameters (see Fig. 3). Furthermore, many beyond Standard Model physics theories, such as sterile neutrinos, non-standard interactions, neutrino decay, etc., have also been constrained by studying possible deviations in the oscillation patterns. With more data and a growing detector, KM3NeT will soon have the world-leading measurements in many of these topics.
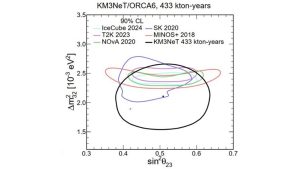
A longer-term physics goal of KM3NeT is to determine the neutrino mass ordering, i.e. whether the third neutrino mass state is heavier (normal ordering) or lighter (inverted ordering) than the first two. This is important to help constrain the plethora of theoretical models proposed to explain the neutrino masses.
Due to the large distances travelled by atmospheric neutrinos as they pass through the Earth’s mantle and core, subtle matter effects come into play and distort the expected oscillation patterns. By comparing the observed distortions with those expected for either the normal or inverted ordering, the mass ordering can be determined to a high confidence level after a few years of operation.
Neutrino astronomy
Weakly interacting with matter and electrically neutral, neutrinos can explore the Universe at greater distances and higher energies than is possible with conventional electromagnetic probes. KM3NeT’s excellent angular resolution and location in the Northern Hemisphere provides an optimal view of the galactic plane and makes it the ideal instrument to detect, characterise and resolve the sources that may emit galactic neutrinos..
Since the observation of a flux of diffuse cosmic high-energy neutrinos in the TeV-PeV range by the IceCube Neutrino Observatory at the South Pole in 2013, the focus of many neutrino astronomers has been to identify the astrophysical origins of these neutrinos. Amongst the diverse possible sources, a multi-messenger astronomy approach has identified the first source: the flaring blazar TXS0506+056. However, the currently identified source population(s) do not fully explain the detected diffuse flux. KM3NeT with a sensitivity superior to that of IceCube and a complementary field of view allows the full neutrino sky to be continuously monitored.
Indeed, recently KM3NeT has reported the detection of a remarkable muon neutrino of exceptionally high energy (see Fig. 4). The neutrino was near horizontal and illuminated one third of the detector, with the energy of the neutrino likely many tens of PeV; significantly higher than any neutrino previously observed.
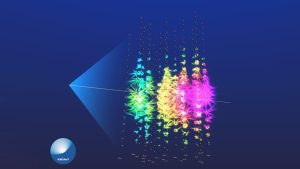
KM3NeT will soon start sending neutrino alerts to its multi-messenger astronomy partners, including electromagnetic wave instruments, other neutrino telescopes and gravitational wave detectors. While so far, no neutrinos have been observed from coalescing binary compact systems detected through gravitational waves, a joint gravitational wave/neutrino detection would reveal unique information on the high-energy processes in the environment of the mergers. Furthermore, the good pointing resolution of KM3NeT significantly reduces the region of interest where observatories should search for an electromagnetic wave counterpart.
Last but not least, KM3NeT is already waiting for the next close-by core-collapse supernova. The KM3NeT optical modules are continuously monitoring for a simultaneous short-duration increase in the amount of light detected on many optical modules — the signature of a flash of MeV supernova neutrinos passing through the detectors. KM3NeT is networked with other neutrino telescopes via the SuperNova Early Warning System (SNEWS).
Deep sea marine observatory
The KM3NeT RI is also a unique deep sea cabled observatory open to all scientific communities, with both detector sites being nodes of EMSO (European Multidisciplinary Seafloor and water column Observatory). The seafloor networks of KM3NeT provide dedicated user ports, to which sensors can be connected, enabling long-term and high sampling frequency monitoring. This is helping marine scientists to understand the harmful effects of climate change and anthropogenic impact, as well as study biodiversity changes, pollution and episodic events such as earthquakes and tsunamis- all of which are difficult to study with short-term conventional marine expeditions
Completion of KM3NeT planned for 2028
The construction of the KM3NeT research infrastructure is well underway and the Collaboration is already providing interesting physics results. Each year it is steadily growing in size and sensitivity as it proceeds towards completion, anticipated for 2028. KM3NeT will then operate for more than 15 years, providing European scientists with a world-class facility to study the neutrino, explore the neutrino Universe as well as the deep sea.
Please note, this article will also appear in the 20th edition of our quarterly publication.