Professor Magda Titirici, Professor of Sustainable Energy Materials at Imperial College, outlines the role of graphite in battery technology and how the latest research is helping to shape and achieve net zero targets.
The British Carbon Group, part of the Materials Chemistry Division at the Royal Society of Chemistry (RSC), is one of many member-driven interest groups to benefit RSC members and the wider chemical science community. Professor Magda Titirici, President of the Materials Chemistry Division at the RSC, is also Professor of Sustainable Energy Materials & Research Director in the Department of Chemical Engineering at Imperial College.
Professor Titirici also holds a fellowship from the Royal Academy of Engineering as Chair in Emerging Sustainable Technologies. This fellowship is for 10 years with the goal of acting as a link/ambassador for different sustainable energy technology developments in the UK. Research in the Titrici Group develops sustainable future energy storage and conversion technologies, such as new battery chemistries that would replace lithium and other critical elements. They are also examining the low-cost production of hydrogen from biowaste with valuable co-chemicals production and fuel cells that are free from precious metal catalysts able to convert hydrogen into electricity in either an acid or alkaline electrolyte.
Lisa Carnwell, Managing Editor at Innovation News Network, spoke to Professor Titirici to understand the importance of graphite as a critical material, the latest battery research trends, and the research taking place to achieve net zero ambitions.
What role does the British Carbon Group play when we consider the science and technology of graphite and other carbon materials?
The BCG’s overall role is to promote and disseminate research concerned with everything related to carbon materials over a wide range of applications. We promote the science or technology properties of graphite and carbon materials, composites, fibres, new forms of carbons, carbon deposits in space, and wider carbon science topics.
The role of carbon as a material is extremely important when we consider renewable energy and the drive toward achieving net zero as it is used in batteries, fuel cells, and electrolysers. However, the BCG also examines the role of graphite for nuclear reactors, carbon in the environment, the potential for bio-applications, and activated carbon for adsorption and gas storage, among a few other topics.
The BCG promotes the UK’s interests and research for carbon materials while many other countries have their own carbon groups, too. Within Europe, most groups operate under the umbrella of the European Carbon Association. They continuously work on disseminating the latest research and information about carbon to enable the implementation of the best and new technologies to foster progress.
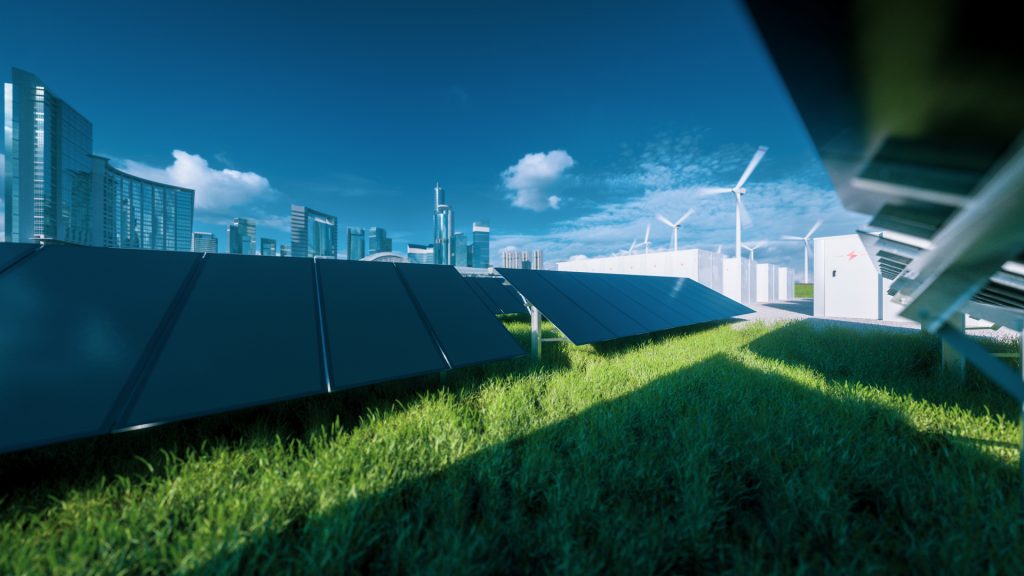
How is graphite used in battery technology, and what applications are available?
Graphite is traditionally used as the negative electrode in a lithium-ion (Li-ion) battery, and since their commercialisation by Sony in 1991, it is safe to say that Li-ion batteries have been integral to enabling us to progress towards the freedom from fossil fuels for power. John B. Goodenough from the University of Texas, M. Stanley Whittingham from Binghamton University, State University of New York, and Akira Yoshino of Meijo University, Nagoya, Japan were awarded the Nobel Prize in Chemistry in 2019 for their discovery which has revolutionised our lives.
There has been huge progress in the development of Li-ion batteries; mostly related to the cathode element with new cathode families out there such as NMC, LMA, etc., but the anode has stayed the same, largely because graphite has a decent capacity (~ 360 mAh) intercalating one lithium per six carbon atoms (LiC6) and cycle performance.
The application range for Li-ion batteries are diverse, from electric vehicles (EVs) to grid energy storage and portable devices. The UK Government committed to ban any new sales of petrol and diesel cars by 2035, encouraging us all to transition towards EVs only. If we want to use the intermittent renewable energy from the wind and Sun, we need grid energy storage. The UK Government has committed to 40 GW of battery storage by offshore wind by 2030 for example, recognising the need for large grid-scale energy storage that batteries can provide. Then, of course, we must not forget all the portable electronics, gadgets, and medical devices etc., where batteries play a key role.
The European Commission has included graphite within its Critical Raw Materials (CRM) list and action plan, but what can the UK and Europe do to secure supply?
Because of the immense demand for Li-ion batteries, manufacturing, and consequently its raw component materials, and because graphite is geographically located in only a few regions of the world (China, India, and Russia for example), graphite is considered by the European Union a critical raw material.
This is clearly problematic for the UK in the light of building the battery gigafactories, as the UK must rely on imports from other countries. China has the largest reserves of graphite and is also the biggest producer of batteries in the world, making it extremely difficult for the UK to compete. In addition, China has also examined the raw materials that it cannot extract for itself and already secured very good deals for all the other critical materials that they need such as cobalt from the Democratic Republic of Congo, lithium from Bolivia, and so on.
It is encouraging that the UK has ambitious targets for battery storage, and I do hope we accomplish them, but at the same time, a clearer strategy for where the raw materials will come from is urgently needed.
China is the largest global supplier of graphite, but there have been new investments for graphite mines in Finland, Sweden, and Norway. Graphite is on the list of critical materials simply because of the huge increase in its economic importance and the demand. Li-ion batteries with graphite anodes will coexist for a very long time with other technologies, but we will see a diversification of the battery markets, using different types of chemistries and different battery technologies where graphite will not be required.
The EU, and in particular some countries like Germany, are doing well in securing access to raw materials. Then, of course, as part of the EU’s policy and strategy for raw materials, the European Innovation Partnership (EIP) on raw materials is a key initiative in promoting innovation in the raw materials sector. As the UK has now exited the EU, it will need to develop its own raw material strategy.
I, along with others, provided evidence to the House of Lords’ Science and Technology Committee in May 2021 on the role of batteries and fuel cells in achieving net zero and the need for a strategy for the UK. The committee has published its report titled Battery strategy goes flat: Net-zero target at risk following the inquiry. The report highlights that unless the government aligns action with their ambitions, the UK not only risks losing its existing automotive industry, but that it will fall even further behind global competitors in battery manufacture, despite the recent announcements of new gigafactories. In addition, we will miss the targets on decarbonisation if we continue to stall our efforts.
Graphite is a material we will most likely need to import, but the UK does now have some access to lithium with mining activities now taking place in Cornwall. I am not an expert on this, but what I understand is that the important aspect is knowing the concentration in the ore. If it is diluted, this will affect the profitability and could make it difficult to extract the lithium from areas such as geological waters. I do not believe the UK will be able to source enough lithium for its own needs from the activities taking place and will still need to import.
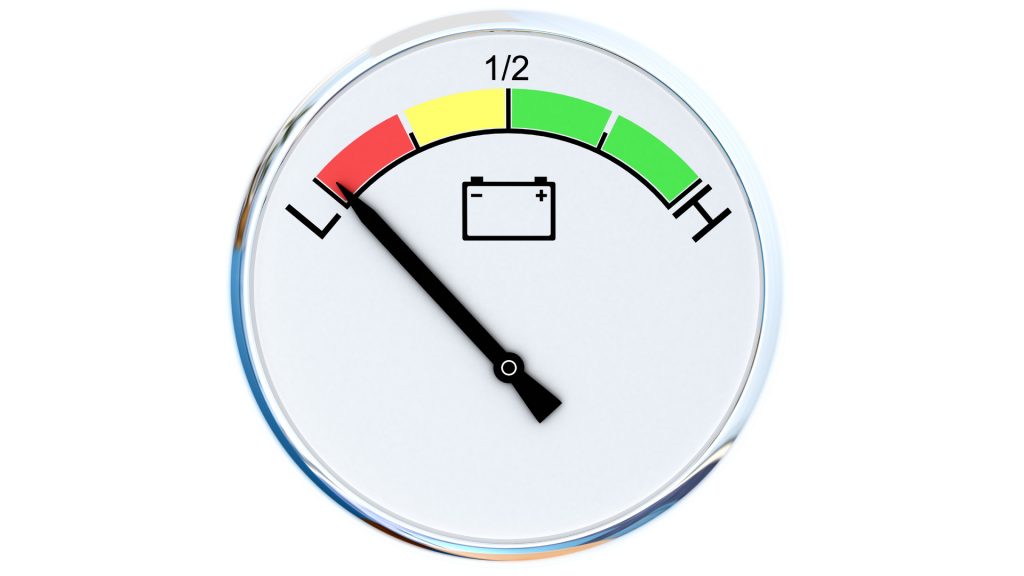
Li-ion batteries with a graphite anode currently dominate the market, yet the huge drive toward battery material recycling is concentrating on the recovery of the very expensive and valuable elements, which are mostly in the cathode
What new research has taken place to increase battery performance in terms of longevity and mitigate against corrosion?
There is a lot of research to mitigate the fact that graphite is a critical element. Of course, there is natural graphite, but you can make synthetic graphite from waste precursors from the petroleum industry such as naphtha, pitches, and tars. Graphite is a specific allotrope of carbon containing sp2 hybridised carbons arranged in a hexagonal structure, which can be created from other carbon forms already containing an aromatic structure with little other heteroatoms, in particular, oxygen. In order to produce synthetic graphite, you must carbonise oil derivatives to exceptionally high temperatures (> 3,500°C) which is very energy consuming. Therefore, the price of synthetic graphite cannot compete with the price of natural graphite. However, it might be an opportunity for the UK oil industries to reorient and align their activities more towards renewable energies and potentially start producing synthetic graphite from these type of precursors.
There is a trend in research efforts in creating synthetic graphite from biowaste and other bio-precursors, but it does not result in perfect graphite and has a very defective structure, unlike that from oil derivatives. These disordered forms of carbon are ideal anodes for alternative technologies such as Na-ion batteries, but they also work to some extent for lithium.
The trends in current battery research are many, but the main goals are to increase performance and the battery life cycle (battery longevity). There is a great deal of research into engineering more efficient cathodes to reach a higher power. Also, preventing the lithium deposition on the graphite anode via dendrite formation is equally important. This can be achieved via understanding battery degradation, understanding engineering the solid electrolyte interface, making batteries more durable, and ultimately going solid state. Replacing the liquid electrolytes with solid electrolytes would prevent such dendrite formations leading to short cuts. However, the challenge here is that you have graphite, which is a solid, and an electrolyte which is also solid, so how do you engineer the interface in between to ensure connectivity? There is a great deal of research in solid state electrolytes and the whole engineering of interfaces.
Research is also oriented towards the better manufacturing of electrodes, eliminating inactive components such as binders and current collectors as much as possible, and increasing the mass of active components. Introducing sensors and active components which can ideally repair batteries during operation is another active and important area of research.
There is also a great deal of research on alternative batteries. So rather than using graphite for Li-ion batteries, researchers are looking at silicon, which could potentially offer a much higher capacity (4,200 mAh/g) instead of 360 mAh/g for graphite. Researchers are also looking at alternative chemistries, so rather than using Li-ion you could use sodium-ion (Na-ion). Na-ion does not need graphite for the negative electrode. This would require a very disordered carbon like the one I described before being produced from biowaste, called hard carbon. This means you do not need to carbonise biowaste to such a high temperature, saving in the overall costs. Researchers are also looking into completely eliminating graphite and instead using metallic lithium or metallic sodium for much higher energy density batteries. I am not saying that we will see the end of graphite use for batteries, but we will see a huge diversification of the battery market and available chemistries. Automation in combination with Machine Learning will also play a major role in this.
Battery technologies have a huge role to play in decarbonising societies globally. The UK Government was the first to set net zero targets, and many companies emerge each year in this space, creating different technologies for batteries. I am sure we will see some electric cars that will still be based on Li-ion graphite, some that will be based on metallic batteries, and some based on other technologies. I think the same will also apply to grid storage and we will see potentially a lot of alternative batteries to lithium such as Na-ion batteries, because they are cheaper.
Graphite faces yet another problem: Li-ion batteries currently dominate the market and have a graphite anode, yet the huge drive toward battery material recycling is concentrating on the recovery of the very expensive and valuable elements, which are mostly in the cathode (mainly cobalt, nickel, and even copper from the current collector). No commercial research efforts towards recycling graphite are happening currently because it is not economically viable. It is also quite difficult to recycle graphite because it is mixed with another type of carbon such as carbon black and carbon from the binder.
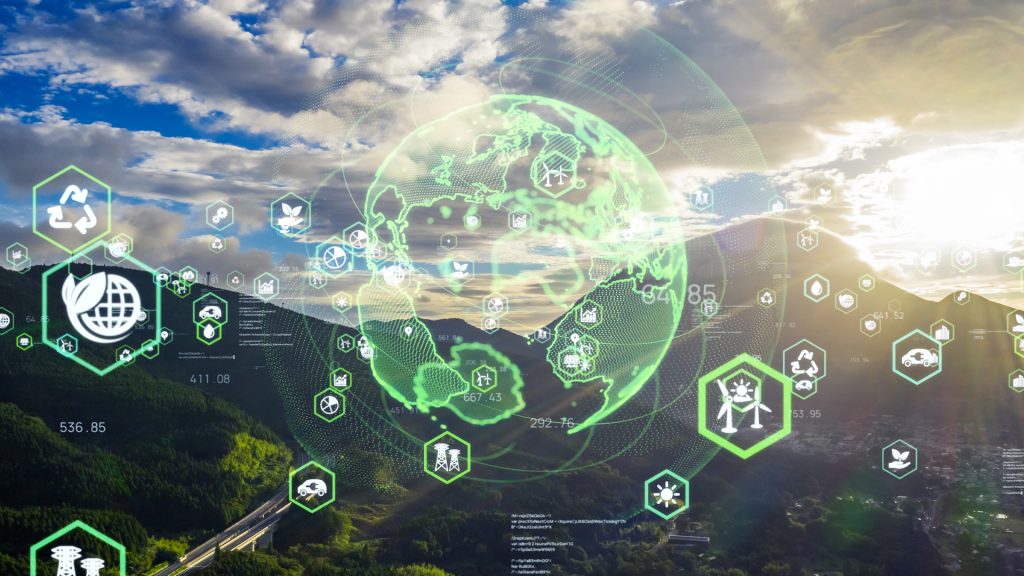
The Transition to Zero Pollution initiative will examine technologies that will enable us to reach zero pollution
Is there any other research that you are particularly excited about, and can you outline where your current focus lies?
There is a lot happening in the battery community. The Faraday Institution, funded by the UK Government, exists to advance battery technologies and make the UK a driving force in batteries. They are pushing some exciting projects, but perhaps one area that I think we are missing is the battery sustainability aspect. Many of The Faraday Institution projects are looking at Na-ion batteries, lithium-sulphur batteries as alternative technologies, solid state batteries, addressing degradation, modelling new cathodes, and recycling of batteries. I think it would be beneficial to see a focused programme on really addressing battery sustainability, including the bottlenecks in the supply chain along with the eco-footprints from manufacturing and recycling. We must think about how we can create the new performance batteries of the future in a sustainable way.
At the European level there is also a great deal of activity, especially with the Battery 2030+ initiative. Their man goal is sustainability and how Artificial Intelligence and Machine Learning could be used to speed up new and sustainable battery discovery.
My research is centred around sustainable carbon materials and applications in energy storage and conversion. Beyond batteries I also work on fuel cells and electrocatalytic applications to produce green hydrogen or reduce CO2 into high-value chemicals or fuels. In batteries, I am particularly interested in making carbon more sustainable and using bio-sources (eliminating really high temperatures) and using more eco-friendly processes. I am really looking at diversifying the market from the commercial products today, so looking beyond Li-ion batteries to Na-ion batteries, potassium-ion batteries, and aluminium-based batteries.
When you think of new materials and new technologies, they take time to develop, but we do not really have the time because we need to get to net zero quickly (by 2050). However, we need to think beyond 2050 and how we can offer long-term sustainable solutions. I think that is why it is crucially important to invest in these technologies now and encourage blue sky research in energy. More research into more sustainable alternatives must be funded.
Another key area is the way we produce hydrogen and we need a body similar to The Faraday Institution that is focused on hydrogen and fuel cells. Again, I would like to see more blue sky research on how we can make hydrogen greener for the future. Simply making it from fossil fuels and adding a bit of carbon capture and storage may help to get us to net zero, but it is not the ultimate solution for the generations to come.
Transitioning to net zero is the ultimate goal, and at Imperial College we take this seriously. Our big initiative, called Transition to Zero Pollution (TZP), is led by Professor Mary Ryan, College Champion, Professor of Materials Science and Nanotechnology, and Vice-Dean for Research in the Faculty of Engineering at Imperial College London. Within this initiative, we are working across all potential technologies to get us to zero pollution. That will include batteries, hydrogen and fuel cells, and everything else that comes with it.
This initiative is built around a cross-disciplinary framework for research based on four pillars of excellence:
- Zero pollution technologies — Using engineering and physical sciences research to develop new zero pollution technologies;
- Environmental health — Better our understanding of air and water pollution, their impact on human health, and how to mitigate them;
- Zero pollution economy — Developing innovative economic and business, policy, and community engagement models for a sustainable society;
- Sustainable water, food, energy — Ensuring that we use water and produce food and energy in a sustainable way; and
- Systems approach to zero pollution — By taking a holistic view of the issues of global pollution we will find solutions to address this major societal challenge.
Professor Magda Titirici
Professor of Sustainable Energy Materials at Imperial College London
President of Materials Chemistry Division at the Royal Society of Chemistry
m.titirici@imperial.ac.uk
Tweet @titiricigroup
britishcarbon.org
www.titiricigroup.com
Please note, this article will also appear in the seventh edition of our quarterly publication.