Professor Stavros Katsanevas, Director of the European Gravitational Observatory, spoke to Innovation News Network‘s International Editor, Clifford Holt, about EGO’s and VIRGO’s role in pushing the frontiers of science, how that is achieved, and the importance of collaboration and co-operation in the field of the experimental and theoretical gravitational waves research in Europe.
VIRGO is an interferometer designed, built, and operated by a collaboration that presently counts 670 members from 125 institutions and 15 different countries with the purpose of detecting gravitational waves as predicted by the general theory of relativity. Working in collaboration with LIGO in the USA, VIRGO helped to make the first such discovery.
The European Gravitational Observatory (EGO) is a consortium designed to ensure the long term scientific exploitation of the Virgo interferometric antenna for gravitational waves detection and to promote research in the field of gravitation in Europe. Amongst its objectives are ensures the functioning of the VIRGO antenna, its maintenance, its operation and any improvements that need to be made and promoting co-operation in the field of the experimental and theoretical gravitational waves research in Europe.
Innovation News Network‘s International Editor, Clifford Holt, spoke to EGO’s Director, Professor Stavros Katsanevas, about both EGO’s and VIRGO’s role in pushing the frontiers of science, how that is achieved, the importance of collaboration and co-operation, and the link between science and art (something that he is keen to develop further).
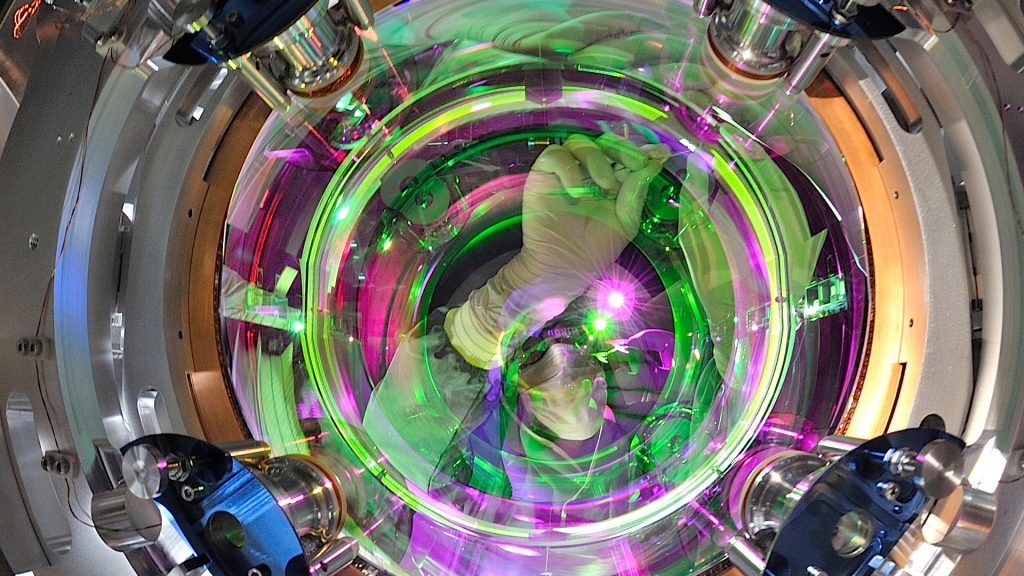
Virgo has been held up as a development as important as when Galileo first pointed his telescope at the sky. Could you describe VIRGO’s journey from inception to now?
In science, patience is crucial, and the detection of gravitational waves – the realisation of which even Einstein was unsure of during the first years (1916 to1918) – was a result of the passion and dedication of those working in the field. Indeed, for some time, we did not know whether gravitational waves could even be detected, and it was only in 1957, after Einstein’s death, that it was realised that we might be able to detect this phenomenon as a change of the length of a standard metre. It took another 60 years to be detected because the metre, which is an interferometer in the case of Virgo and LIGO, must be protected from every other source of noise, and so a very complex system needs to be developed to achieve that. In the process, we also needed to develop a whole series of other technologies – from stable lasers to ultra-reflective and low loss large mirrors. Concerning Virgo, a first evaluation report was published in 1990, and in 1993-4 the project was approved. Construction of the VIRGO facility began in 1997 and in 2000 EGO, the consortium that essentially hosts the facility, was established.
The Virgo science round started in 2007, and we soon realised that a global network was required because, if we want to see where something in the sky comes from, then we need to triangulate the signal. From 2007, LIGO and VIRGO have therefore operated as a single machine – and last year we were also joined by KAGRA; we are a worldwide gravitational wave network.
In September 2015, the US interferometer (LIGO) made the first detection of gravitational waves: the merger of two black holes that happened 1.4 billion light years ago. It is important to note that although, at the time, the Virgo antenna had not yet entered into operation, the discovery happened in the context of the LIGO-Virgo scientific collaboration.
LIGO’s second observation run since being upgraded in a programme called ‘Advanced LIGO’ began in the summer of 2017. A month later, in August, the Virgo antenna also completed its upgrade and entered into the network. Two weeks later, the Virgo and LIGO interferometers detected another gravitational wave signal from the merger of two black holes. The fact that there were three antennas that made the discovery allowed a ‘triangulation’ and therefore the identification of the region of the sky where the event occurred with unprecedented precision.
A few days later, on 17 August 2017, another type of event was detected: a neutron star merger triggered a follow-up campaign with about a hundred observatories detecting signals using electromagnetic ‘messengers’ ranging from high energy photons to visible light and radio waves. The concomitant observations of the merger in electromagnetic and gravitational waves were thus the inaugural event of what is now termed ‘multi-messenger astronomy’, since it permits the exploration of the Universe not only with light or, more generally, with electromagnetic radiation, but also with gravitational waves, deeply probing the time evolution of the Universe’s violent events. It is important to note that for this last detection, on 3 February 2021, Virgo and the two LIGOs were dedicated as a historical milestone by the worldwide Institute of Electrical and Electronic Engineers (IEEE).
Since 2019 and the new round of observations, we have been making an average of one detection per week. And, of course, new upgrades have been approved which will increase this to one detection per day. In the longer term we hope to increase this further still, to one detection every few hours, making gravitational wave antennas comparable with the electromagnetic observatories where we see new events all the time.
Moving forwards, some of us are also involved in developing the Einstein telescope, a third-generation, gravitational-wave observatory, which we have very high hopes for.
How does the collaboration with LIGO and KAGRA work?
We are all a part of the common network that we established ourselves, and the management teams meet every Tuesday to discuss how we are going to continue to work together. One of the main things we have identified is the need to share alerts and information more quickly, and we are discussing ways to achieve that, as well as how we can ensure that this information is standardised.
A second important point concerns computing power. If we are to achieve our goal of making new detections every hour – or even more frequently – then we need to be able to analyse the data, and that presents numerous challenges. To overcome those hurdles, we will need to work together.
In terms of the experiments themselves, we have had to build larger, better quality mirrors, and we have been co-ordinating the R&D on this because we are so sensitive that even the movement of atoms in the mirror can have an unwanted effect. In the future, we therefore believe we will have to use cryogenic temperatures and perhaps even new materials. We are now working together to overcome the challenges that arise from trying to achieve these temperatures while simultaneously firing a laser at the mirrors, which heats them up.
We are also working together to explore how we can extend the bandwidth to low frequencies, where the main noise comes from the environment.
In addition to the mirrors about the low frequency frontier, a third area where we need to work together is in what might be termed ‘the second quantum revolution’. That is, we have a laser, and the precision we can obtain in these frequencies is determined by the power of the laser (that is, how many photons there are). Characteristically, the square root of the number of the laser photons gives you the noise level, which we need to control before we can begin to understand how to be sensitive to higher frequencies. We do that by employing a technique known as ‘quantum squeezing’, which requires keeping the process in quantum ‘entanglement’ – just as in other quantum techniques for sensors in quantum computing.
We freely share what we do in all of these areas between the observatories because we know that challenges such as better sensitivity, better computing, and low latency alert distribution require co-operation rather than competition, and we are developing synergies to achieve that.
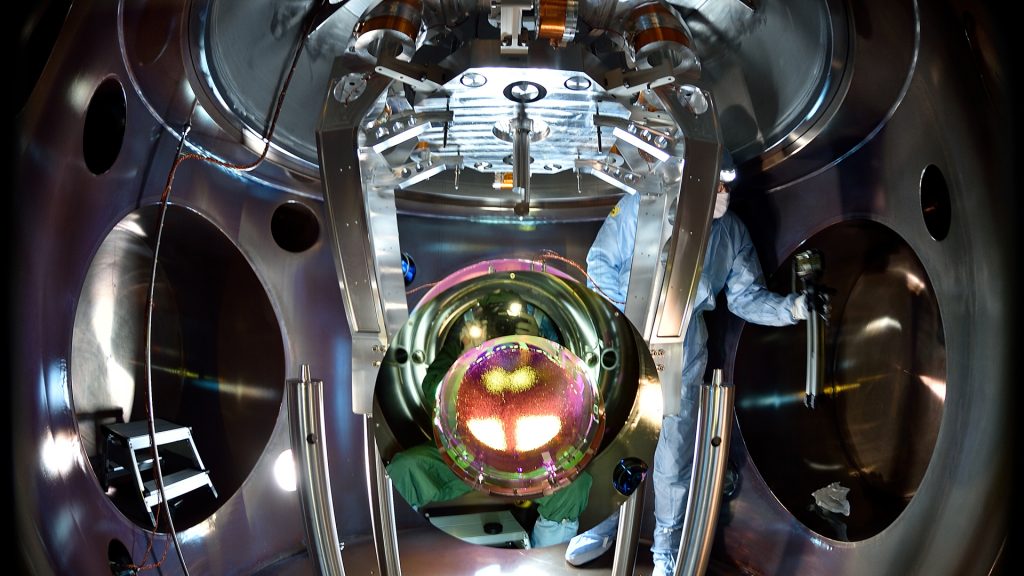
The detection of an electromagnetic counterpart to gravitational waves gave birth to what is now known as multimessenger astronomy, as you have mentioned. How has this field since begun to evolve? Why is it important? How important will Virgo/LIGO continue to be, moving forwards?
In 1987, we observed the light from a supernova explosion (now known as SN 1987a), but we also detected it through the neutrinos that it emitted, although it was not until afterwards that we discovered the synchronicity. This was before the terms ‘astroparticle physics’, which attempts to mix particle physics and astronomy, had been properly developed, of course. Indeed, at this time there was some resistance from both sides – some astrophysicists felt as though they were being invaded by particle physicists, and some particle physicists saw those attempting to work on astroparticle physics as deserters; it took some time for the interdisciplinary approach to be properly accepted. Now, of course, most people understand that the messages from the Universe are multiple – and so we need multiple approaches and multiple disciplines to see and hear them.
The different messengers enable you to probe different parts of the process. Neutrinos come from deep inside the process, while electromagnetic waves come from the surface, for instance. So, by measuring the different things we can gain a better understanding of what happens after the explosion, and we can follow the process from the beginning, just seconds after it happened millions and millions of years ago, to the time when the radio waves start to arrive. This is therefore the first time that we are able to study the processes of the Universe in all of their phases, rather than simply developing simulations.
This also enables the development of different cartographies of the Universe. We all have the image of the Universe that is enabled from the position of the stars – which may be termed the ‘living’ objects in the Universe – in the night sky. But, of course, that light has often taken millions of years to reach us, and so we are looking at the Universe as it was at that time; when we use light to create an image of the Universe, it is not the Universe of today.
When we measure gravitational waves, we measure what might be termed the ‘dead’ things in the Universe – black holes, neutron stars, and even white dwarfs; the end products of stars that have completed their lifetime. This enables us to create a new, different cartography that maps how stars behave when they have finished their life. These two cartographies can then be compared, and every difference holds the promise of new physics. This comparison helps us to better understand how the stars were created and how they evolved, and there will also be things that are missing – for instance, questions remain about the nature of dark matter and dark energy. By creating the different cartographies in relation to the different messengers, and by comparing the maps, we can find differences, and each difference provides a hint of where you have to go to better understand what exists in the Universe.
While the potential cannot be understated, the challenge lies in being able to co-ordinate our activities, and on 20 to 24 September, in Florence, we are hoping to organise a workshop to gather as many people as we can across disciplines to try to figure this out. We also want to ensure that the data we collect on the Universe’s many signals, from the radio to the very high energies to the slowest possible messengers, is publicly available. This is crucial if we are to be able to put out alerts with sufficient time for the different telescopes to be able to turn to the point of interest and contribute to the observations. It is needless to say, that the development of technology to distribute early warnings and alerts has applications that extend beyond the strict domain of science, particularly in these times of climate change and worldwide connectivity.
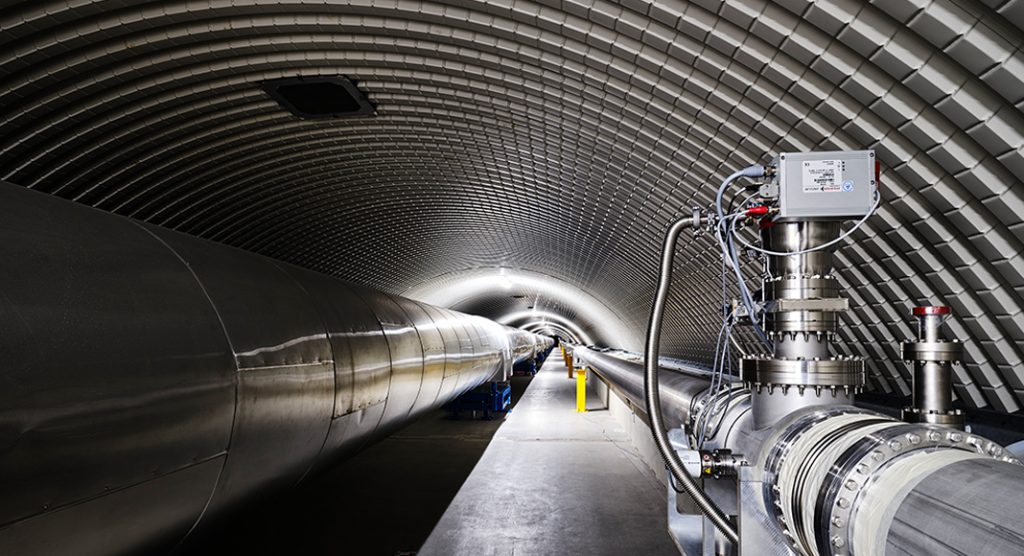
LIGO’s second observation run since being upgraded in a programme called ‘Advanced LIGO’ began in the summer of 2017
What are your thoughts on how ESA’s LISA and ATHENA missions – as well as perhaps the JWST – will help this field to develop? What would you like to see this new field discover in the future?
With LISA, we would be able to see two objects – such as black holes or neutron stars – spiralling around each other months or even years before they coalesce; we will be able to see the event coming by using the multimessenger paradigm to predict when the event will happen ahead of time so that all the relevant observatories can turn their attention to it.
In addition, LISA will also address a bandwidth much lower than the sonic – at 0.1 to 100 mHz. In many instances of measurements across multiple bandwidths, many of the degeneracies of the signal are raised. Again, this will enable LISA to see the events before and as they happen; and it will be able to determine the exact masses and exactly what happened on the frontier. ‘Multi-band’ observations (space and Earth) provide very important information. For instance, they allow us to explore whether the black holes being observed have ‘hair’ (that is, in opposition to the Einstein–Maxwell equations of gravitation and electromagnetism in general relativity, they have retained some information about their past), or what the equation of state is for any neutron stars being observed, and so on. To obtain this information, it is necessary to conduct multiband astronomy – observing at both the low and high frequencies.
Alongside this, we are also putting together proposals to locate gravitational wave detectors on the surface of the Moon, which would aid us significantly in triangulation activities.
Athena and JWST will probe the violent events, e.g. jets created during the mergers, in X-rays and the infrared, and will therefore help us follow the process in its evolution, and also contribute significantly to the future of multimessenger astronomy via the combination of photons and X-rays.
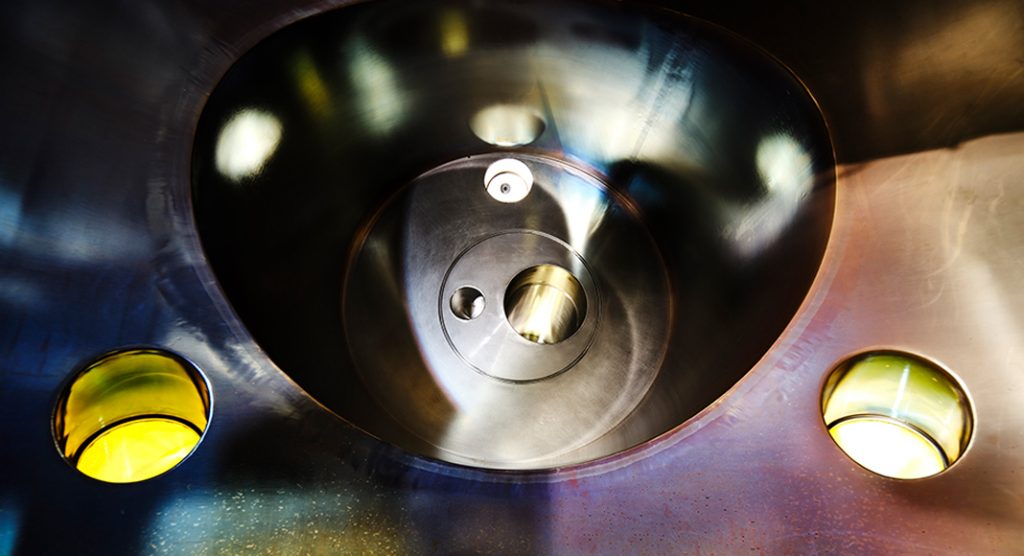
We have talked about how the discovery of gravitational waves has been compared to the work of Galileo. Can we also expect this discovery to have an impact on education, art, and society in the post-pandemic era, just as it did in Galileo’s time?
This is a very interesting subject, and at EGO we have a significant programme, which is supported by the EU, to achieve that.
We know that many of Galileo’s scientific discoveries and ideas found their way into the art of the time. Indeed, numerous books have been written on the subject. Galileo and his work had a direct influence on the perspective in paintings – such as in Lodovico Cigoli’s version of the Assumption of the Virgin, which depicts the Moon as though being viewed through a telescope, for instance – with Galileo having published Siderius Nuncius just two years earlier, which included a very similar image. And the same art, of course, also impacted Galileo, while he also had an impact on music.
The same kind of movement happened at the beginning of the 20th century, when the new geometries were discovered and Einstein developed his theory of general relativity, which had an impact on the work of artists such as Picasso, Duchamp, and others.
There has always been a link between art and science. As Frank Oppenheimer famously said, artists and scientists are society’s ‘noticers’; they observe things that others miss, and while scientists work on the rational side and artists on the more affective side, we are two sides of the same coin and are united by the idea of metaphor – in the sense of, say, the thought experiments of Einstein and Galileo on the scientists’ side, and the imbedded spaces of imagination of the painter on the artists’ side.
We want this to be realised once more, and we are involved in several collaborations to achieve that. For instance, at one of our events the artist Tomas Saraceno streamed his concert ‘How to hear the universe in a spider web’, that included the sonorisation of gravitational wave events. That is incredibly interesting because the frequencies we are attempting to measure with the interferometric signal of are, of course, a luminous signal rather than sound. And yet the frequencies are up to 10 kilohertz, which is the acoustic boundary, and so it is often said that we are able to ‘hear’ the Universe.
Our multimessenger observations have indeed allowed us to view the Universe with more than just one ‘sense’, as it were; we can ‘see’ it via the electromagnetic waves; we can ‘hear’ it by translating the vibrations of spacetime itself into acoustic signals; and we may also argue that, because particles such as neutrinos and cosmic ray particles enter into our detectors in the same way as the olfactory system works, we are also able to ‘smell’ the Universe. We are thus developing a multisensorial understanding of the cosmos.
This brings us to some of the activities that we have already begun in terms of the sonification of astronomical data.
Wanda Merced Diaz, an expert on the sonification of astrophysical data, recently joined the EGO staff. However, we do not want this to simply be an exercise that is just ‘illustrative’, ‘interesting’, or ‘fascinating’ but which has no real merit or impact; we want to include it as a core element of the work that we do moving forwards because, as we increase our perceptual capabilities, our understanding will also improve.
Such new and innovative approaches also tend to help develop new perspectives, especially in terms of individuality; we hope to be able to better understand the different ways that people see the world and perhaps even evolve new ideas about spacetime. Indeed, it is important to acknowledge that spacetime is not something that exists only in the far reaches of the Universe; you are experiencing it when you are in a city; an artist experiences it when he is painting; a geologist experiences it during her fieldwork. We are interested in how people see this.
We are also in contact with Saul Perlmutter, who was awarded the Nobel Prize in Physics 2011, and whose ‘Big ideas Berkeley’ critical-thinking course, ‘Sense and Sensibility in Science’, inspired our REINFORCE (Research Infrastructures FOR citizens in Europe) project.
You have frequently said that the main task is to isolate the interferometer from environmental noise, and that inversely this effort becomes an opportunity to study the environment in return. Can you elaborate on this?
Because of our incredible sensitivity, Earth’s natural seismicity, anthropogenic movements, electromagnetic variations, and even the mass of a cloud passing overhead can affect the position of the mirrors, and so we need to collaborate in interdisciplinary consortia in order to gather data on atmospherics and geosciences, which we utilise in our gravitational wave experiments. We have a programme at EGO designed to do just that. There is a natural synergy between these scientific areas, and we are frequently entering into a number of collaborations with those working in the geosciences.
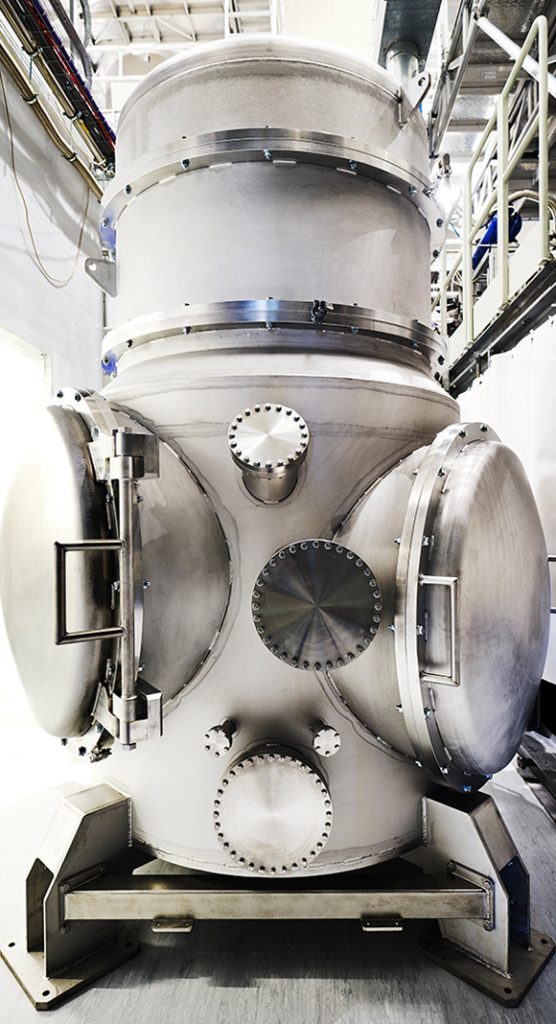
The fourth LIGO-Virgo-KAGRA observing-period has been postponed. How significant is this? And what are your hopes for the future, once observations commence once more?
We have been fortunate not to have been too severely impacted by the COVID-19 pandemic – we were forced to close for a month and, while we were to begin the next round in January 2022, we have had to postpone that for a total of six months. But, given the situation, things could have been a lot worse.
Moving forward, we want, as we have discussed, to increase the number of events that we see. In addition to the signals from the coalescence of two objects such as black holes or neutron stars that can be seen with our detectors, the signal from the rotation of objects such as neutron stars can also be detected at LIGO and Virgo. And while we have not yet been able to achieve that, we hope to be able to do so in the future, and that will provide us with a lot of very important information.
We would also like to observe a burst from a supernova; but that depends a lot on luck. If we were to actually see a supernova together with neutrinos, then I expect to also detect gravitational waves, and that would be a fantastic achievement. Unfortunately, these are rare events – occurring about three times a century in the Milky Way – and it has been some 40 years since the last one, which means we need to be lucky if we are to see the next as we have no way of knowing where and when it will happen.
Finally, we want to ‘hear’ the ‘sound’ of the Big Bang. To achieve that, however, we need extreme sensitivities, and this will therefore take some time.
We have already been able to observe black holes in regions where we were not expecting to find them, and so, alongside the pre-defined science goals, we are also looking forward to the unexpected, whatever that might be.
Professor Stavros Katsanevas
Director
European Gravitational Observatory (EGO)
stavros.katsanevas@ego-gw.it
Tweet @ego_virgo
www.ego-gw.it/
Please note, this article will also appear in the sixth edition of our quarterly publication.