Dr Matteo Guainazzi, Study Scientist for the European Space Agency’s Athena mission, spoke to The Innovation Platform’s Clifford Holt about some of the ways that the mission will probe the hot and energetic Universe.
IN 2014, Athena – Advanced Telescope for High-ENergy Astrophysics – was selected as the second large (L-class) mission in ESA’s Cosmic Vision programme. Scheduled for launch in 2032, it will combine a large X-ray telescope with state-of-the-art scientific instruments and will map and study large-scale gas structures in the Universe, survey supermassive black holes, and explore high-energy astrophysical events such as supernova explosions and energetic stellar flares.
Dr Matteo Guainazzi, Study Scientist for the European Space Agency’s Athena mission, spoke to The Innovation Platform’s International Editor, Clifford Holt, about some of the mission’s instruments and how it will use them to probe the hot and energetic Universe.
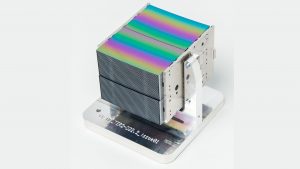
What is the background to the Athena mission? How does it build upon the successes of past X-ray missions?
Europe has been at the forefront of X-ray astronomy for decades (since the time of Exosat). Athena picks up on the success of XMM-Newton which, in terms of published scientific papers over the last decade, has been ESA’s most productive and successful mission (although it was recently overtaken by Gaia). It covers a wide spectrum of different celestial sources, from the X-ray emissions of planets and comets to the farthest object in the Universe, including galaxies and their accreting black holes.
Typically, when a mission such as XMM-Newton has been such a success, the scientific community tries to imagine in which observational parameters a new mission can exceed it by at least one order of magnitude. This would allow the new mission to do revolutionary science, and so the community also begins to think about what kind of innovative and transformational science could be achieved.
Athena’s goal, quantitatively speaking, is to improve on the performance of XMM-Newton by at least one order of magnitude in terms of collecting area and spectral capabilities.
The key payload feature of Athena is its mirror; this will be the largest X-ray mirror ever built in the history of astronomy and will deliver a collecting area at least 70 times larger than that of any other combination of mirror plus spectrometer, when compared to both existing and planned missions. This mirror required completely new technology to be developed as its mass also needs to be sufficiently low for it to be launched on a spacecraft – it weighs two tonnes and is filled with 600 individual modules that are able to focus the X-rays and with an accuracy several times better than XMM-Newton.
Athena will carry a completely innovative payload, including a cryogenic X-ray spectrometer called the ‘X-ray Integral Field Unit’ (X-IFU) which will essentially measure the tiny changes in the temperature that are induced by X-ray photons in a superconductive material. This is a completely new technique that will allow us to measure the spectra of celestial sources with an accuracy an order of magnitude larger than any existing mission. The X-IFU is able to measure the spectrum of a celestial source on each individual pixel. It will have over 3,000 of these pixels, each with a size of about five arcseconds. This means that we will be able to measure the physical properties of hot (i.e., million degrees) astrophysical plasmas, such as their density, velocity, temperature, metallicities, and so on, on the spatial scale of just a few arcseconds which, for X-ray astronomy, is a significant achievement and has never been done before.
Furthermore, Athena will carry a Wide Field Imager (WFI), which will have a 40’x40′ field of view. This instrument will scan a certain number of pre-defined positions in the sky, and it will detect an enormous number of mostly unknown X-ray sources thanks to the unprecedented combination of effective area and field-of-view, providing moderate-resolution spectra for most of them. This instrument will allow us to study and characterise statistically representative spectral samples of X-ray emitting sources.
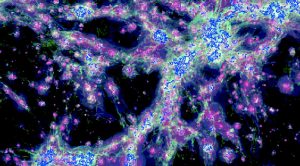
One of the main things that Athena will explore is the formation and evolution of galaxy groups and clusters. How will this be achieved?
We know that the Universe is not homogeneous. If you look at scales of gigaparsecs – 1,000 times larger than the size of a typical galaxy – this becomes immediately apparent. We also know that the Universe is structured in filaments, what is called the ‘cosmic web’, and most of the gas in these filaments has been heated to millions of degrees (and is therefore actually a plasma, rather than a gas). When a gas becomes so hot, it primarily emits X-rays. As such, if you want to study the structure of the Universe, and more specifically if you want to know how the temperature, metallicity, and content of the Universe evolved over time, then you need to look at these structures in X-rays.
Galaxy clusters are the largest gravitationally-bound structures in the Universe, and they are located at the points where these filaments meet – there is an increase in density here, and this is where matter collapses to form groups and clusters of galaxies. These are the areas that Athena will explore as they are the regions where it is possible to probe the plasma’s physical properties. What is more, it will be possible to do so from distances that correspond to the cosmological time when we believe these structures were forming, through to the current Universe. While studying the evolution of the Universe’s metallicity is an interesting topic in itself, it also reveals much about the entire lifecycle of stars, as each and every element in the Universe was born out of a certain type of supernova explosion.
Athena is also set to shed light on the ‘missing baryon’ problem. How will this be approached?
The plasma in the cosmic web’s filaments has to be very dense for it to be visible, and that is why we look at galaxy clusters, as this is where the density is highest. However, even in regions where the densities may be not sufficient a priori for emissions to be visible, you can still conduct experiments as there are very bright X-ray sources in the background. The radiation that reaches us from such sources crosses through these filaments of hot gas, which absorb some of it. As such, the missing baryons (which we believe could be one phase of the hot filaments) can be detected through the absorption lines they create in the spectrum of a background source. This background source could, for instance, be a gamma-ray burst, the most powerful explosion in the Universe, and Athena has the unique capability to quickly (within a few hours) point to such an event, thereby observing it before it decays and becomes invisible. There are also permanent bright sources located between us and the filaments of hot gas, and Athena will survey over 100 of them over its nominal operational life.
This is currently a somewhat controversial topic, however, and as yet it is unclear whether evidence of these missing baryons has been detected by ESA’s XMM Newton and NASA’s Chandra. If it is indeed the case that much of the missing baryons are in the strands of gas that make up the warm-hot intergalactic medium, Athena will be able to see them, and ultimately probe their existence.
Similarly, how will Athena help to better understand the evolution of supermassive black holes?
All galaxies host a ‘super-massive black hole’ in their centre, with a mass million to billions the mass of the Sun. About 10% of these galaxies shine in X-rays. The black hole is the reason why these galaxies are so X-ray bright, as the matter that accretes around a black hole is heated to extremely high temperatures and is also accelerated to very high speeds, while the magnetic fields close to black holes are extremely strong. These are the conditions needed for matter to become sufficiently energetic that it is able to emit X-rays.
Many of the black holes we are be able to currently detect are relatively close by. However, Athena will be able to detect hundreds of accreting black holes down to the time when the Universe was only about one (out of thirteen) billion years old. We will be able to measure their spectrum and therefore decipher the properties of the X-ray emissions. Through this, we can study the black hole’s evolution.
We also know that such black holes not only accrete matter very rapidly, but they also eject matter in jets and winds with very high relativistic speeds. It is thought that these outflows have an important impact on the evolution of the host galaxy, as the stream of matter ejected from the black hole heats the interstellar medium, thereby depositing energy and momentum on the matter in the galaxy, potentially moving the plasma beyond the borders of the galaxy itself, or heating the gas to such high temperatures that collapse to form new stars becomes impossible. Indeed, black holes could regulate star formation through feedback mediated by powerful outflows. Studying them through the background of the black hole X-ray emission is the only way to characterise their properties, because the plasma in the outflows is constituted primarily of highly ionised ions, and therefore absorbs X-ray photons.
How will Athena contribute to the evolving field of multi-messenger astronomy?
We know that there is at least one electromagnetic counterpart to a gravitational wave event, and we hope to be able to use Athena to observe the merging of two neutron stars in X-rays – assuming, of course, that their electromagnetic emission is as predicted by theory.
Through this observation, we will be able to do two things. Firstly, we will be able to directly observe the jets or streams of relativistic particles that are emitted when the neutron stars merge and a strong gamma ray burst is produced. These jets will be important because they essentially tell us about how the matter surrounding the neutron star merger event is accelerated. They will also allow us to probe the metallicity and other physical properties of the interstellar medium where these events occur. Secondly, by observing (in X-rays) the light curve from the remnant of the merged neutron stars, we may be able to tell whether this remnant is a neutron star or a black hole. This is important to the theories that study the evolution of neutron stars and black holes from explosive merging events of this kind.
In addition, an exciting possibility is that the Athena and LISA missions may fly together. This could enable Athena to also observe the electromagnetic counterparts of merging supermassive black holes. This will be extremely interesting because, prior to the black holes merging, we might be able to see how the matter accreting around them is affected by the changing spacetime structure, meaning that we may be able to test predictions of how matter is expected to behave in the strong gravity regime of general relativity. And, once the merger has occurred, we could also witness the birth of a new active galactic nuclei (AGN), a new accreting black hole.
What stage has the mission now reached? What challenges have you encountered so far? Are you on schedule to begin construction?
We have entered into Phase B1 following the achievement of a robust, convincing design for the mission as a whole. This will involve looking at the different elements in more detail and will end in June 2022, with the formal adoption of the mission, after which point we will be able to begin construction.
Dr Matteo Guainazzi
Study Scientist
Athena mission
European Space Agency
Tweet @esa
Tweet @esascience
matteo.guainazzi@sciops.esa.int
www.cosmos.esa.int/web/athena
Please note, this article will also appear in the fourth edition of our new quarterly publication.