Neil Ward, Vice President and General Manager at PacBio, outlines the potential of genomic sequencing to understand and address threats to wildlife.
The past few decades have seen biodiversity decline at an alarming rate, stemming from human activities like land use change and habitat pollution, combined with the effects of climate change.
Subsequently, global biodiversity intactness has fallen to 75%, markedly under the agreed safe limit of 90% for warding off an ecological recession. Wildlife in the UK is equally as vulnerable; since 1970, the abundance of UK priority species has declined by 60%, making it the worst of the G7 countries.
But there is hope. Innovations in biodiversity genomics have the potential to help scientists address threats to biodiversity. With highly accurate genomic sequencing, researchers gain molecular-level insights into organisms, so they can investigate species’ health and population dynamics. Such granular data powers early and targeted action for endangered species, including conservation strategies such as breeding programmes and reforesting initiatives.
How to unravel biodiversity with genomics
Biodiversity genomics helps researchers understand why some species thrive, and to uncover warning signals that suggest a population may soon be in decline. Here is a brief overview of how this research works:
1. Capturing and cataloguing species
Biodiversity research begins by building reference genomes. These references capture and catalogue species to demonstrate how ecosystems operate and locate any dependencies. To build high-quality reference genomes, researchers must consider the read length of sequences. There are two main approaches, short- and long-read.
Short-read has previously been the most popular method and involves breaking the genome into small fragments and then piecing them back together.
However, this process is intricate and can lead to errors or an incomplete picture of an organism, especially for species with complex genomes. Choosing long-reads to build reference genomes improves accuracy and completeness, since DNA is sequenced in larger segments. A jigsaw with a few large pieces is much easier to put together than one with many small pieces that all look similar.
Researchers should also consider a multiomic approach, including data from the genome, proteome, transcriptome, and epigenome in references. DNA doesn’t often tell the whole story, so the other ‘omes’ add more dimension to better understand underlying biological connections and associations with risk factors like disease.
2. Identify traits for adaptation
Once reference genomes are assembled, scientists can use the data to answer crucial questions about how species are evolving. Reference genomes help identify which genes are most likely to cause physical changes and which genetic variations are most dominant.
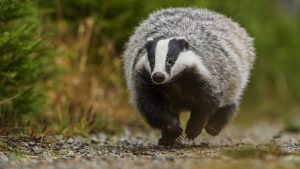
For example, sequencing can unravel why the badger is more vulnerable to tuberculosis, or why red squirrels are susceptible to leprosy. Such insights can help inform breeding programmes by pinpointing plants and animals with more favourable adaptations.
3. Understand population diversity
Understanding the level of genetic diversity in a population allows researchers to predict which species are at risk of decline. Populations with high genetic diversity are more likely to survive new environmental conditions, whereas species with low genetic diversity are less likely to adapt, increasing the risk of extinction.
Scientists know to prioritise intervention in that area if genetic variation declines in a local population.
Sequencing the UK’s wildlife with Darwin Tree of Life
One example of a DNA-driven conservation project is The Darwin Tree of Life, which is working on the genomic sequencing of all 70,000 species on the British Isles. By building detailed reference genomes, researchers are monitoring the evolution of the UK’s ecosystems to support biological research and aid conservation.
The project chose to use the latest, most advanced long-read sequencing technology to afford researchers the deepest and most accurate possible insights into species, especially those with more complex biology.
The Darwin Project has already sequenced many species, including:
- The killer whale (Orca) – Killer whales have a genome about 96% of the size of the human genome. British populations can be small and highly inbred, making them prone to ill health. By understanding the orca genome, researchers can identify health problems earlier and help local populations to thrive.
- Freshwater alga – This species produces around one-third of all oxygen in the atmosphere and locks away enormous amounts of carbon dioxide. With a greater understanding of its genome, researchers hope to harness its ability to confine carbon dioxide, and produce more efficient biofuels.
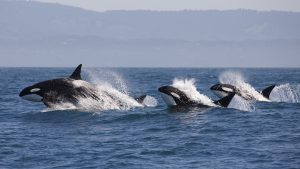
How genomic sequencing technology shapes the next era of conservation
The Darwin Tree of Life project is one of many collaborative partnerships deepening our understanding of wildlife and shaping conservation – but there is still a long way to go. Despite advances in scientific research, an estimated 80% of the world’s species still await scientific discovery and description. Even for described species, telling them apart can often be challenging without biological insight.
To realise the promise of genomics in biodiversity, there must be greater uptake of complete and accurate sequencing technology in wildlife projects, giving researchers the full picture of genetic variation in nature. Advances in long-read sequencing have made such technology increasingly accessible, bringing us one step closer to scaling in-depth biodiversity research.
Long-read sequencing systems are more affordable today and can now deliver more genomes per year, with reduced sample sizes and exceptional accuracy. By harnessing the latest sequencing technology, researchers can better understand the ecology and conservation needs of species before they are lost.