Building on the legacy of predecessor PHENIX, a new RHIC detector, sPHENIX is set to release the first results that will shine new light on the structure of the quark gluon plasma.
With the advent of the Relativistic Heavy Ion Collider (RHIC) a quarter century ago, the field of Relativistic Heavy Ion finally had access to a fuller suite of signatures of the long searched-for new state of nuclear matter known as the quark gluon plasma (QGP).
Specifically with RHIC’s high collider centre of mass energy of 200 GeV per nucleon-nucleon collision, a plethora of hard scattering probes which could be reliably calculated by perturbative Quantum Chromodynamics (pQCD) could be put to bear on the remnants of RHIC’s Au+Au collisions.
Amongst the most anticipated of these were the highest energy probes, jets and the particles that made them up or were produced with them, such as photons. Jets, which are single quarks or gluons knocked out of the initial colliding nucleons, ‘hadronise’ into many particles, all moving close by the original particle’s trajectory. These can be used to penetrate the collision remnants, providing both an ‘x-ray’ and also ‘sampler’ of the QGP matter distribution.
After just its first five years, a concert of positive results, including several of the jet-related QGP signatures, led to the definitive confirmation of RHIC’s discovery of the QGP in 2005.
An important contributor to the heavy ion scientific community’s confidence at the time and confirmations in subsequent years were RHIC’s competing and dual confirmations of its two largest experiments, STAR and PHENIX. PHENIX was designed with a two-fold focus on the hard-scattered, penetrating probes: an extremely high-precision and fast Electromagnetic Calorimeter and an extremely high-rate readout electronics and Data Acquisition (DAQ) system capable of measuring and sampling photon and electron final-state particles at a much faster rate that complemented the originally charge-particle-only sensitive tracking focus, and lower event rate capability of the STAR detector.
Through the use of these systems, especially the calorimeter, many of the key QGP-signature measurements were led by the PHENIX, including the hardest scattered jet probes at that time measured by particles with the highest-at-RHIC final state pT, momenta transverse to the initial incoming nuclear momenta (and therefore coming completely from scattering). These showed an expected suppression called jet-quenching due to jets being attenuated by the QGP and losing significant energies while traversing it.
However, not everything about the QGP and its formation was completely understood. Although quark and gluon degrees of freedom were clearly observed at some probing scales, there were still questions about whether extra structure might exist in its constituents when probed across all length scales and at all temperatures that the plasma existed in during its evolution in each event.
Further, by the early part of the 2010s, a complementary but even higher beam energy Relativistic Heavy Ion programme at the Large Hadron Collider (LHC) was taking jet-related measurements to new levels of precision and complexity using detectors that were more optimised for jet measurements than the RHIC experiments. These measurements further hinted at the extra complexities and introduced new opportunities.
In order to facilitate the pursuit of these opportunities at RHIC and fully realise a direct comparison between the RHIC vs LHC plasma collision evolutions with side-by-side measurements of the same quantities measured the same way, PHENIX was recently replaced by a new detector, sPHENIX, modelled more directly on the LHC detector advantages. sPHENIX took its first physics dataset this year and is set to shed new light on QGP physics.
Jet and photon–related quark gluon plasma signatures brightly illuminated by the PHENIX EMCal
Its quark and gluon nature itself causes photons which are produced with the QGP to play a special role in its study. Measurements of these were amongst those that prominently allowed the very high-quality EMCal of PHENIX to shine.
Firstly, the majority of particles produced in RHIC collisions by the decay of the QGP state, up to several thousand in a single event, as well as those produced by the jets themselves, are the lightest quark bound state, the pion, of which a third are neutral (so-called π0 mesons) and decay immediately to two high-energy photons.
The PHENIX EMCal subdetectors used fast detection processes, firing over timescales less than a nanosecond typically, making for particularly good ‘triggers’ for sampling extremely high event rates to filter out only the relatively rare large energy hard scattering events in real time. This allowed the PHENIX measurements to collect more of the very highest pT. π0‘s allowing the study of jet quenching to the highest jet energies at RHIC with single particle measurements.
Secondly, because of the high temperature of the QGP, literally, the hottest substance known to exist in the universe at roughly 4 trillion Kelvin, a large number of ‘black-body’-like thermally radiated photons are radiated, and PHENIX was the first to use these to measure this temperature with its EMCal.
Finally, to extract even more information about jet modification by the QGP, a complimentary ‘golden channel’ for studying the jets was also well-suited for PHENIX’s EM Calorimetry. The jets are most often produced in pairs (‘di-jets’), with each member of the pair having equal pT, but after both potentially traverse the QGP, both can potentially be modified, resulting in uncertainty on the original energies of either jet.
By instead studying jets produced via a competing process where one of the jets is replaced by a single ‘direct’ photon, also known as a ‘prompt’ photon (see Fig. 1), one avoids this uncertainty since/ the photon is electrically neutral and not sensitive to QCD colour charge, is unmodified allowing for a calibration for the jet produced with it. PHENIX was the first to confirm that the prompt photon cross section was indeed not suppressed like the jets, which at the same time confirmed the whole ‘Glauber’ theoretical framework used to predict the pre-QGP rates of all hard scattering probes, including the jet cross sections themselves that made interpretation of the jets’ suppression to be unambiguous.
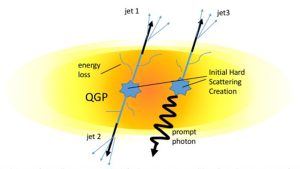
In later datasets, PHENIX also made many of the key measurements of this channel through correlations of hadrons produced by the jets of the photon-jets, using the two-particle correlation (2PC) method, including, for example, showing that the lost energy of the associated jets was reappearing in the form of many more low energy hadrons produced very close but in a region around the jet.
The LHC era ushered new precision and opportunities in jet and photon-related physics
The first results of the heavy ion programme at the LHC (LHC HI) early in RHIC’s second decade already showed the difference the newer and larger-sensitive area detectors of the LHC could make. Two of these, ATLAS and CMS, were designed primarily for more exacting High Energy Physics (HEP) jet measurements at much higher jet energies than those accessible at RHIC.
Further, at these energies, pQCD theory guidance is even more reliable, leading to a revolution in jet-related observables. The thrust of the LHC HI jet measurements was using methods of fully reconstructing the jets from their many-particle constituents, a method first attempted at RHIC by STAR just before the start of the LHC era, but that proved challenging for STAR and PHENIX due in large part to detector insensitivities.
In addition, the before-mentioned questions about the structure of the plasma began to emerge, including what were the proper constituent degrees of freedom relevant at different scattering resolutions and during the QGP’s formation in each event. LHC data and related theory revealed many new insights, such as that natural fluctuations unrelated to QGP in the structure of the jets themselves could affect the energy loss differently.
All the while, during the last half of RHIC’s lifetime, continual improvements to its own accelerator performance and luminosity meant that RHIC could finally achieve comparable statistics in the very high energy (and exponentially rarer) jet energies at the low end of the LHC’s reach and thereby a good overlap of measurements of the same jets kinematically as LHC.
This set the stage for direct comparisons of LHC vs future RHIC datasets in the same-energy observables. Such comparisons would be especially fruitful since the different collision energies cause different time and temperature evolutions for RHIC vs the LHC plasma creation in each event.
Necessary for this was an improved detector that could function more like the LHC detectors to make such direct comparisons possible. With this opportunity in mind, PHENIX stopped data-taking in 2016 so that its detector hall could house the new detector sPHENIX whose construction and installation lasted from around 2018 to 2023.
sPHENIX: A new detector at RHIC optimised for jet and photon measurements
From its initial design, sPHENIX, shown in Fig. 2, was optimised for jet measurements. A key part of ensuring this optimisation was also consistent with the tradition of PHENIX was designing sPHENIX to have excellent and expanded calorimetry. Built around the recycled cylindrical solenoid magnet formerly used in the BaBar experiment at SLAC, which was capable of stronger magnetic fields, the sPHENIX EMCal was built extremely compactly with a cylindrical radius of only one meter (compared to 5m for sPHENIX) from the beam collision point. This allows the EMCal to fit inside the magnet in order to significantly increase the sensitive angular acceptance area while maintaining high precision with minimum noise-causing materials in front of it.
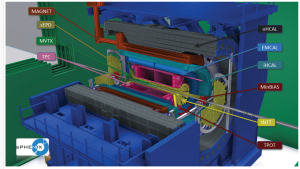
The EMCal was also made with finely segmented sub-units designed with a unique projective geometry that makes their sensitivity extremely constant across the whole detector. This feature is very important for fully reconstructing all the daughter particles of each jet, which will generally span many of these individual subdetector elements because this reduces possible misinterpretation of simple detector non-uniformities being mistaken for the expected QGP-induced modifications to only certain parts of a single jet’s elements.
Having kept an excellent EMCal in the new sPHENIX detector is also especially important for sPHENIX’s goal of making comparable measurements with the LHC because of an extra benefit of the extended high energy reach at RHIC in the golden photon-jets due to a coincidence in the energy dependence of the production cross-sections of the prompt photons, the backgrounds for the golden photon-jet events at RHIC are much smaller than at LHC and further extend the benefits of making the LHC-RHIC comparisons in this important channel.
In addition to its strong EMcal, which can greatly improve upon the measurements of photon-related measurements, another new feature of sPHENIX, never before employed at RHIC, is its other high-quality calorimetry system, a full set of hadronic calorimeters. Hadronic calorimetry is an important part of the highest quality LHC jet measurements made by ATLAS and CMS because it catches the sizable portion of the jet constituent hadrons that are neutral but not photon-decaying and cannot be detected; neither the electromagnetic calorimetry nor charged particle tracking, previously the only options employed by both PHENIX and STAR.
With this additional capability, as well as a suite of high-precision charged tracking detectors, including an innovative, compact Time Projection Chamber (TPC), the optimisation of sPHENIX is made complete.
In spring 2023, the installation of sPHENIX was completed, and a dedicated commissioning run of RHIC was made to bring sPHENIX online and calibrate all of its subsystems. Commissioning of the detector was completed over the subsequent year, with the first physics quality data being taken with the fully functioning detector, and initial calibrations were completed starting in the summer of 2024.
The 2024 RHIC running which followed was mostly focused on a large p+p dataset at the 200 GeV centre of mass energy that will be used mostly as the control dataset for a longer Au+Au dataset at this energy scheduled for 2025. The RHIC collider performed at a high level as expected, and sPHENIX recorded a very large p+p dataset, sampling twice as many p+p events as originally planned for its final results.
In addition, the first physics quality Au+Au dataset with total recorded statistics for hard-scattering physics is comparable to most previous PHENIX datasets. The first analyses of this data are currently underway and slated to have the first official results released for the Quark Matter 2025 conference in April 2025. This should be the first taste of what will prove to be interesting in the next several years while sPHENIX realises its physics mission to shed new light on the QGP by making direct comparisons with the LHC jet and photon measurements. This will be the penultimate realisation of RHIC’s legacy of its addition of jet and photon hard scattering into the worldwide quest to understand and characterise the QGP.
Please note, this article will also appear in the 21st edition of our quarterly publication.