Finding empirical evidence for life on planets outside the Solar System has profound implications for understanding our own origins here on Earth.
Life is everywhere on Earth. It fills the air we breathe in the form of microorganisms and has spread into every corner and niche we can imagine, including deep inside Earth’s oceanic crust at the bottom of the sea. Life has been around for about four billion years (which is roughly 90% of Earth’s lifetime), and, interestingly, all lifeforms we know on Earth share the same principles for some of their fundamental functions, indicating that all lifeforms are related and can be traced back to a universal common ancestor. Yet, it is still unknown as to where, when, and how life began. Similarly, whether it truly arose only once, giving rise to the widespread but single kind of life we see today, seems unlikely but is unclear. In short, we do not understand the origin of life and cannot say whether it was a lucky chain of random events happening under very specific conditions or whether it was a logical and natural consequence of standard planetary evolution.
Co-ordinated efforts to address the origin of life
Over the last few years, many universities and research institutions started co-ordinated efforts – in the form of interdisciplinary networks or research centres – addressing from the perspective of natural sciences where we (and all other lifeforms on Earth) are coming from. A recent example is the new ‘Centre for Origin and Prevalence of Life’ that was established at ETH Zurich.1
One of the main drivers for these centres was the realisation that investigating the origin(s) of life requires a truly cross-disciplinary approach. A single discipline alone lacks sufficient knowledge and expertise, given the complexity of the challenge. A standard example is the question of the formation of complex organic molecules, such as RNA and its precursors, which are considered a vital step towards the formation of life: while it is the goal of pre-biotic chemistry to reveal via which formation pathways and reaction networks and with which basic ingredients these molecules can be formed, it is crucial to consider the ambient conditions on early Earth under which these reactions might have taken place. What was the temperature and pressure of the Earth’s early atmosphere? What was its composition? What was the pH level of the immediate environment on the surface? Chemists typically do not concern themselves with these questions, but they are of immediate relevance if one wants to make sure that the right boundary conditions for origin-of-life experiments are used.
The role of astrophysics
At ETH Zurich, but also in other centres and research networks, astrophysicists are strongly involved in the research activities as they can contribute important pieces of the puzzle. For instance, there is the question of the radiation environment of the young Earth: how much radiation was provided by the young Sun and how much ended up on the Earth’s surface, as opposed to being shielded by the atmosphere? Of particular importance here is the high-energy flux provided by the ultraviolet part of the Solar spectrum. These photons are a unique and potentially plentiful source of energy that can trigger chemical reactions that would otherwise not take place. However, because they are so energetic, the photons can also break apart molecules and prevent the formation of more complex compounds. In addition, astrophysics comes into play when it is about understanding the formation pathway of planetary systems and the delivery of fundamental chemical building blocks that were available on the young Earth. Next to investigating the leftovers from the Solar System’s formation phase, such as comets and asteroids, revealing the (chemical) composition and the physical properties of planet-forming circumstellar disks around young stars is a major line of research in that context.
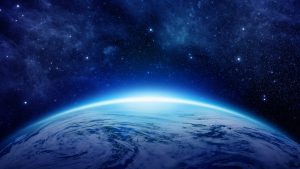
Beyond the obvious: The role of exoplanet science
While these activities link directly to the question of the origin of life here on Earth, exoplanet science also has an important role that at first sight may not be so obvious. With more than 5,0002 exoplanets (planets orbiting stars other than our Sun) already known today, the in-depth characterisation of these objects is becoming increasingly important – and technologically feasible – in addition to discovering more extrasolar worlds.
In fact, one of the big hopes for the James Webb Space Telescope (JWST) is that it can help us understand if small, terrestrial exoplanets, objects with sizes and masses similar to Earth, that orbit low luminosity, but highly active, cool dwarf stars are able to retain an atmosphere despite the stars’ strong high-energy radiation output. However, a detailed atmospheric characterisation of these objects, i.e., generating an overview of the atmospheric main constituents, will likely be beyond JWST’s capabilities. In the long run, however, this is exactly one of the main goals of exoplanet science: being able to determine the atmospheric composition of dozens of Earth-like exoplanets. The reason is that the atmosphere encodes critical information about the planetary environment and – even more importantly in the context of origin-of-life research – it can also contain spectral features that indicate the presence of a biosphere on a planet. Hence, by being able to determine what exoplanet atmospheres are made of, we have a chance to identify on which planets life may exist. For example, oxygen, which is produced by plants and algae ( i.e., life) makes up roughly 21% of Earth’s atmosphere today. For an external observer from a remote vantage point, it leaves detectable imprints in the form of so-called atmospheric absorption bands. As such, the detection of oxygen in the atmosphere of an exoplanet could hint towards the existence of life.
Next to oxygen, there are also other gases, such as methane or nitrous oxide, which are considered good candidates for so-called atmospheric biosignatures, and a number of research groups try to understand which gases can be added to this list. A critical aspect of this work is to investigate what abiotic processes could also lead to the accumulation of a significant amount of these gases in a planetary atmosphere mimicking the signal caused by biological activity. In many cases, the simultaneous detection of pairs of biosignatures that effectively hint at a strong chemical disequilibrium, such as having oxygen and methane at the same time, is considered the most robust and reliable signal to date.
But how does this relate to the origin of life? Currently, our home planet of Earth is the only place in the Universe that is known to host life. Finding indications of life on another planet orbiting another star could provide first evidence that, instead of origin of life, we are actually dealing with origins of life and that life might be much more widely spread. This could indicate that pathways and processes leading from non-living matter to living entities are robust and more universally applicable and not uniquely constrained to the specific conditions found on early Earth. This implication would be particularly important if the star-planet systems, for which such inferences were made, were very different from the Sun-Earth system in terms of fundamental properties such as masses, temperatures, and compositions.
The LIFE mission
It is in this context where the LIFE mission3 is no longer seen as the next (or another) exoplanet space mission. As shown in a series of peer-reviewed scientific papers4,5,6,7,8, LIFE will be able to directly detect dozens of Earth-like exoplanets around different types of host stars, characterise their atmospheric composition, and search for biosignatures. Hence, if done right, the scope of the LIFE mission and its possible scientific heritage expands well beyond astrophysics and touches upon one of the most fundamental questions of humankind. Therefore, joining forces with other disciplines to help identify what atmospheric signals to look for, and under what circumstances such signals provide strong evidence for biological activity, is key. Also, comprehensive statistical frameworks that allow researchers to quantify these statements will need to be further developed and validated. Even more importantly, however, as we are increasing our understanding of how life may have arisen on Earth, we will be able to start formulating hypotheses for which stars and planet types it is more (or less) likely to find indications for extra-terrestrial life using life as we know it – and that is all we have – as a reference. Work in this direction has already started in some of the collaborations mentioned above, effectively bridging between many scientific disciplines. Still, more systematic efforts are needed. The results will be directly fed into the activities of the LIFE initiative so that the chances that LIFE can be fundamentally transformative in our understanding of the origins of life are maximised.
References
- www.copl.ethz.ch
- https://exoplanetarchive.ipac.caltech.edu
- www.life-space-mission.com
- https://arxiv.org/abs/2101.07500
- https://arxiv.org/abs/2112.02054
- https://arxiv.org/abs/2204.10041
- https://arxiv.org/abs/2210.01782
- https://arxiv.org/abs/2211.04975
Please note, this article will also appear in the twelfth edition of our quarterly publication.