Bora Gencturk, Associate Professor from the University of Southern California, summarises the findings from various studies looking at storage and transportation issues for spent nuclear fuel.
Clean electricity generation through nuclear power plants (NPP) is facing a key turning point due to issues surrounding the spent nuclear fuel (SNF) storage. The typical fuel for NPP is in the form of enriched uranium pellets that are stacked inside a cylindrical fuel rod. The fuel rods are combined with other elements required for the fission reactions in fuel assemblies consisting of tens of rods. SNF assemblies are hot and radioactive, they are considered high-level nuclear waste, and they require proper handling and storage. The waste remains radioactive for hundreds of thousands of years.
Dry cask storage for spent nuclear fuel
The primary storage solution for SNF is inside large fuel pools located in a fuel handling facility within the NPP station. The limited space in the fuel pools led to the development of additional temporary storage known as dry casks. Three to five years of cooling in the fuel pools allows for a sufficient decrease in the temperature for the transfer of SNF into the dry casks. Several SNF assemblies are packaged inside a cylindrical stainless steel canister, which is then placed inside an overpack for additional radiation and thermal shielding. A typical dry cask is 3-4m in diameter, 5-6m in height, can weigh up to 140 t, and hold close to 90 SNF assemblies. Tens of dry casks are placed in a grid on a concrete pad in fuel storage installations.
If the US is taken as an example, the continuing growth of SNF at a rate of 2,000-2,300 MT per year with no permanent storage solution led to an extended use of dry casks beyond their initially intended service lives.
As a temporary solution, the first dry cask was licensed in 1986 at the Surry Nuclear Power Plant in Virginia. Without a solution for permanent storage, after 35 years, there are 90 independent spent fuel storage installations (ISFSI) in the US as of April 2021. The first ISFSI has been in service for nearly 40 years and is approaching the end of the second 20-year license period. However, the interim storage period of SNFs is still indefinite. An analysis of dry casks for up to 300 years has been considered. Thus, the long-term service of dry casks is bringing significant challenges in terms of aging evaluation and management, including radiation shielding, containment and structural safety, and heat removal capacity. The prolonged lifetime of these structures also increases the probability of them being subjected to lower frequency events.
The issues stated above have led to two main considerations. One is related to the performance of the dry cask overpack and the canister, in terms of maintaining their integrity to continuously provide thermal and radiation shielding. The second is related to the integrity of the package contents (nuclear waste, in this case) prior to and after transportation to an interim or permanent storage facility. Numerous studies have been conducted on both topics in the past two decades, in addition to earlier destructive tests performed on dry casks in the 1970s and 1980s considering various accident scenarios such as drop, fire, ballistic impact, and crash.
Assessing the long-term performance of temporary SNF storage solutions
In this article, I will discuss the key findings from the studies performed by my colleagues and I over the past decade through projects funded by the U.S. Department of Energy and the Nuclear Regulatory Commission.
We performed accelerated aging of 1/3-scaled reinforced concrete overpacks, followed by tip-over impact experiments. Two aging mechanisms were considered separately in different physical specimens: namely, the corrosion of the steel reinforcement inside the concrete and the alkali-silica reactivity (ASR) of concrete. The latter mechanism is known as the concrete cancer and results in the internal cracking of concrete slowly over time, leading to degradation in the mechanical and physical properties. The tip-over impact is a potential incident that could occur due to overturning caused by an event such as an earthquake or ballistic impact. It was found that, despite some damage to the overpack (especially when combined with corrosion or ASR), the canister maintained its integrity.
Our estimates indicate that the amount of aging due to corrosion in accelerated laboratory conditions corresponded with 75-300 years of service life for a prototype cask in different environmental conditions representing different locations within the US. Earthquake risk analysis performed for extreme low values of friction coefficient between the cask and the concrete pad revealed that the probability of exceeding a predefined sliding limit state of a quarter of the diameter of the cask and the probability of overturning are on the order of 10-5 and 10-6 for locations in western, central, and eastern US with known seismicity. These results indicate that typical casks are well overdesigned for their intended service life for conditions considered and can possibly be used for periods exceeding 100 years, if proper aging management programmes are put in place.
In separate studies, we considered different accident conditions through computer simulations. As mentioned previously, these accident conditions become more relevant as the dry casks are used for extended periods of time. We simulated the performance of reinforced concrete and steel-concrete-steel sandwich cask overpacks under near-field blast loading. When the stresses in the canister lid and the radial deformation of the overpack were used as measures, no major physical damage was found to the canister for blast charges of up to 25kg equivalent of TNT at a detonation distance of 5m. Similar studies were conducted for extreme impact loading simulating an aircraft crash into a dry cask. We found that the lateral impact of a 4.4 t aircraft engine at a speed of 150m/s to the top of the cask might cause a tip over for casks that are not secured to the concrete pad through bolts.
We found that a mid-height impact causes a larger deformation to the canister and the closest SNF assemblies, as the top and bottom of the cask are stiffened by the presence of the lid and the base plate. The steel-concrete-steel sandwich overpack configuration was more effective in terms of reducing the penetration depth and increasing the cohesiveness of the structure impacted by projectiles. Simulation of a 9m horizontal drop event showed a minimum cladding margin to failure of 20% for the highest loaded fuel rod in the assembly. Integrity of the rod cladding (i.e., the tube that encapsulates the fuel pellets) is essential as cladding failure could lead to the release of fission gases into the canister cavity. These results should be interpreted within the context of the probability of such an event occurring. Like the sliding and tip-over probabilities mentioned above, the probability of such a low altitude impact of a large aircraft engine or 9m horizontal drop event is very low.
Transportation safety of commercial spent nuclear fuel
With regards to the second issue of transportation safety, these dry storage canisters will need to be moved to a ‘more permanent’ location at some point in time. Since transportation in large numbers has not been undertaken before within the US, there are lingering questions regarding the safety of transportation.
Our second research thrust has been on the topic of integrity assessment of SNF canisters using non-destructive evaluation (NDE) methods. Even if a fuel handling facility is available, opening and inspecting these packages is very costly, aggravated by the fact that thousands of these packages exist. We have been working on three separate NDE methods that can provide complementary information about the package contents. These methods are the time-tagged neutron interrogation, elastic dynamic analysis, and acoustic sensing. Before dwelling on these methods, I would like to also mention that our most detailed analyses indicate that, even in the worst case scenario, SNF stored in a canister does not become critical (i.e., uncontrolled chain reaction leading to a meltdown event).
Time-tagged neutron interrogation
In the time-tagged neutron interrogation, fast neutrons and gamma rays emitted as a result of the interactions of the primary beam with the SNF were detected and used as a signature to identify missing fuel rods. We found that fast neutron measurements are a viable approach to detect missing pins inside a fuel cask that could, for example, be the case due to damage from normal reactor operations, storage or an accident during storage or transportation. We were able to identify and locate at least 50% of the pins removed with an overall measurement time of less than two hours and a source strength of at least 1010 neutrons/s in the 4π solid angle using organic scintillation detectors. The simulated inspection time of less than two hours is much shorter when compared to other proposed methods such as muon tomography, which may require an inspection time longer than 24 hours.
Elastic dynamic analysis
For the elastic dynamic analysis, we created arguably the most detailed computer model of a canister consisting of over 130 million degrees of freedom. It is not feasible to solve such a large model even on the most powerful supercomputers within a reasonable time frame. Therefore, we developed efficient solution methods that enabled us to perform probabilistic damage assessment purely based on vibration measurements on the exterior surface of the canister. We were able to accurately detect the presence and location of damage inside the canister including missing or degraded fuel assemblies.
Using probabilistic Bayesian model updating, we could detect and locate even minute damage scenarios, such as degraded attachments between the fuel rods and the fuel assembly structure or the fuel assemblies and the fuel basket. We verified the feasibility of this approach on a 2/3-scale SNF fuel canister with 68 fuel assemblies. Using measurements collected on the bottom plate of the canister, we could determine the location of missing fuel assemblies. Work is ongoing to extend this technology and implement it in real SNF canisters.
Acoustic sensing
In acoustic sensing, the main principle is to send a sound signal through the cavity of the canister (usually filled with pressurised helium) and detect it on the other side to determine the time of flight of the sound wave. Like the previous two methods, the sensors are external to the canister and measurements are only collected from the outside.
Detecting changes to the gas composition is of great significance for canister monitoring. The gas properties may change during prolonged storage. For instance, xenon (Xe) can be introduced to the backfilled helium when the fuel rod cladding is compromised. If the canister is breached and leakage occurs, the helium pressure could decrease, and the temperature can increase because of reduced helium conduction and convection. In addition, water vapour may also be mixed with helium due to insufficient vacuum drying. These abnormalities could indicate potential problems within the canister.
Therefore, the backfilled helium property can be a useful indicator of the conditions of the fuel, the canister, or both. The challenge in this approach is to couple the sound wave to the gas medium due to very large acoustic impedance difference between the steel and the gas. A large amplitude sound wave travels through the steel and overshadows the air signal. To overcome this issue, we have developed noise cancellation methods to silence the wave travelling through the steel. With proper optimisation, we were able to achieve a 90% reduction in the noise. This work is still ongoing – we are further refining and extending this method to render it applicable to a real SNF canister.
Finding a permanent SNF storage solution
Finally, I would like to add a few words on the efforts moving towards a permanent storage solution for SNF. At the time of writing this article, there is no permanent storage solution. Certain countries, such as France, Japan, Russia, and the UK, partially reprocess the SNF, which reduces the amount available for storage. France, Germany, Hungary, Sweden, Switzerland and Ukraine have operational central storage facilities. The US has recently made efforts towards partly centralised ‘interim storage facilities’. The biggest leap forward has been the licensing and ongoing construction of the first ever deep geological repository (DGR) in Olkiuoto, Finland, which is expected to become operational around 2025.
In DGRs, the SNF is encapsulated in robust and leak-tight containers and buried several hundred metres below ground in a system of tunnels. Being several hundred metres underground isolates the radioactive material from potential more dynamic near-surface geological events for hundreds of thousands of years, needed for the radioactivity in the waste to decay. Several other countries are considering similar DGRs. The US has long had plans for a similar DGR, which has not moved forward due to political and other reasons. Successful implementation and proliferation of DGRs will definitely make clean energy generation through nuclear power a more viable and attractive option.
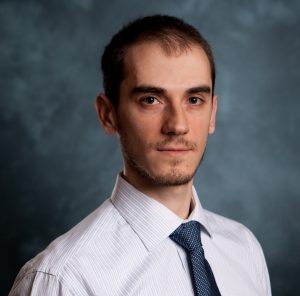
Acknowledgement
I would like to conclude by acknowledging my co-investigators and numerous students who have contributed to the work summarised above. This work was done in collaboration with several universities and national laboratories in the US.