Dr David YW Ng and Professor Dr Tanja Weil from the Max Planck Institute for Polymer Research, outline how their lab has designed a new peptide capable of changing the physical structure of cancer cells.
The treatment of cancer via chemical drugs dates back to the 1940s with the discovery of nitrogen mustard, by Goodman and Gilman,1 to induce tumour regression. The scientists subsequently elucidated the molecular action of the mustard compound and found that it is transformed into an alkylating agent capable changing the physical structure of cancer cells by crosslinking them. Following this success, the development of chemotherapeutics accelerated in the following decades with the discovery of anti-folates,2 topoisomerase inhibitors3 which are still used as the first line of chemotherapy regiment in many malignancies.
While there is no doubt that these FDA-approved regiments and combination therapeutic strategies have saved countless lives, frustrating problems with side effects and cancer resistance/resurgence continue to overwhelm scientists and physicians. Hence, the modern era of chemotherapy has evolved into targeted approaches where biologicals such as antibodies are recruited to enhance both the potency and selectivity of anti-cancer agents.4 Despite the increased variety and constant refinement of anti-cancer agents, solutions for metastatic and drug resistant cancers remain unfortunately elusive.
Our laboratory at the Max Planck Institute for Polymer Research in Germany has therefore decided to take a leap of faith by exploring new mechanisms to target and destroy cancer cells.
Conventionally, anti-cancer drugs work by inhibiting or modifying a biological target that is crucial for cancer proliferation and thereby achieving therapeutic outcomes.1 In contrast, our research team, headed by Professor Tanja Weil and Dr David Ng, invented a peptide drug that infiltrates the cancer cells and grows into artificial fibre networks from within, killing the cells outright by disrupting their structural integrity.5 The transformation of the non-toxic peptide drug into fibres is controlled and switched on by conditions present in cancer cells (see Fig. 1).
Applying strategies from Alzheimer’s disease to cancer cells
The technology developed in our laboratory is inspired by how several neurodegenerative diseases decimate brain cells with tangles and webs made up of insoluble protein fragments.6 The accumulation of these fragments cannot be overcome by any biological means, be it through biodegradation or extrusion. The result is the spread of acute toxicity of these tangles on neurons without any possibility of treatment.
Re-engineered by Michaela Pieszka, a Ph.D. scientist in the team, the principles behind the tangle formation are spliced into small peptides and chemically overhauled. Molecular attachments and triggers were designed to safeguard the peptide from assembling into tangles under normal physiological environments. Upon entry into cancer cells, the increased level of reactive oxygen species such as hydrogen peroxide activates the trigger, initiating a series of chemical transformations of the peptide. As the safeguards fail, the peptides begin to congregate and assemble into insoluble fragments, which propagate into tangles and fibre networks, all within the cancer cell. We have demonstrated that these networks change the physical structure of cancer cells where their presence caused the cytoskeleton to break down (see Fig. 2).
The cytoskeleton is an important cellular organ where it is both the primary structure element of cells as well as acting as transport highways. As the disrupting fibre networks cannot be overcome by any biological arsenal of the cancer cells, a cellular suicide process (apoptosis) is initiated. Reminiscent of Alzheimer’s disease, the causative agent is the assembly of peptides into insoluble fibres that breaks apart crucial biological connections which forces cells to die.
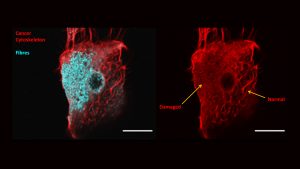
© 2020, Max Planck Institute for Polymer Research
The Tetris® Design Principle
The design of the peptide follows a principle based on molecular geometry. Resembling a game of Tetris®, a straight (l) block has multiple possibilities to stack together perfectly, compared to a (T) block which has only a single possibility. Similarly, a linear (l) short peptide sequence has multiple ways to connect multiple copies of itself, allowing it to grow into bundles, forming stable networks and fibres. On the contrary, a (T) shaped peptide sequence has not only a minimal chance of stacking in a correct way, and any budding assemblies will also be too unstable to propagate in the biological environment.
Hence, by using a chemical technique developed in our laboratory, we can specifically programme a T shaped peptide to be transformed into an l peptide within cancer cells (see Fig. 1). As cancer cells generally metabolise at a much faster rate, the by-products such as reactive oxygen molecules like hydrogen peroxide are produced at a higher level than normal cells. Therefore, we took advantage of the elevated hydrogen peroxide levels to trigger the peptide transformation and the subsequent production of fibre networks.
Outlook and perspective
The technology developed in our laboratory was demonstrated at the cellular level with a proof of principle published in the Journal of the American Chemical Society.5 It has received immense interest in international news and social media. Future development entails several parallel studies including:
- Animal models
- A next generation design that offers biodegradation after cancer cells die
- Expanding the technology to drug-resistant cancers
References
- Chabner, B., Roberts, T. ‘Chemotherapy and the war on cancer’. Nat Rev Cancer 5, 65–72 (2005)
- Jolivet, J., Cowan, K. H., Curt, G. A., Clendeninn, N. J. & Chabner, B. A. ‘The pharmacology and clinical use of methotrexate’. N. Engl. J. Med. 309, 1094–104 (1983)
- Saltz, L. B. et al. ‘Irinotecan plus fluorouracil and leucovorin for metastatic colorectal cancer. Irinotecan Study Group’. N. Engl. J. Med. 343, 905–914 (2000)
- Scott, A., Wolchok, J. & Old, L. ‘Antibody therapy of cancer’. Nat Rev Cancer 12, 278–287 (2012)
- Pieszka, M., Han, S., Volkmann, C., Graf, R., Lieberwirth, I., Landfester, K., Ng, Y.W.D., Weil, T. ‘Controlled Supramolecular Assembly Inside Living Cells by Sequential Multistaged Chemical Reactions’. J. Am. Chem. Soc. 142, 15780–15789 (2020)
- Gan, L., Cookson, M.R., Petrucelli, L. et al. ‘Converging pathways in neurodegeneration, from genetics to mechanisms’. Nat Neurosci 21, 1300–1309 (2018)
Dr David Y.W. Ng
Group Leader (Synthesis of Macromolecules)
Professor Dr Tanja Weil
Director (Synthesis of Macromolecules)
Max Planck Institute for Polymer Research
david.ng@mpip-mainz.mpg.de
weil@mpip-mainz.mpg.de
www.mpip-mainz.mpg.de/en/weil/groups/ng
www.mpip-mainz.mpg.de/en/weil
Please note, this article will also appear in the fourth edition of our new quarterly publication.