Osteoclasts have a vital role as cells responsible for the continuous resorption of bone matrix. The question thus arises as to why the adequate therapy of many diseases caused by increased osteoclast activity is still a major challenge.
For all vertebrates, bone tissue has, in addition to biomechanical properties for mobility and stability or organ protection against external influences, also other diverse, vital functions in mineral metabolism and blood formation. Our bone tissue is subject to a continuous recycling process by building (anabolic) cells (osteoblasts) and decomposing (catabolic) cells (osteoclasts), whose interaction is regulated in a highly complex way, thus ensuring a dynamic balance between both of them. Many organ-related diseases are caused by a disbalance of this equilibrium and vice versa. Osteoclast-related diseases include osteoporosis, periprosthetic osteolysis (PPOL)1, rheumatoid arthritis, bone tumours, Paget’s disease, and osteopetrosis.
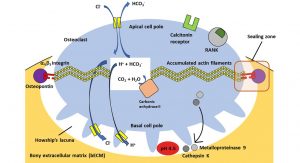
The catabolic osteoclast and its role in bone metabolism
The mineralised substance of human bone is, as in all vertebrates, a molecular composite consisting of proteins and biomineral elements and contains four differentiated, bone-specific and localised cell types: osteoblasts, bone lining cells, osteocytes, and osteoclasts. We do not want to go into detail here about whether bone lining cells are ultimately also osteoblasts (epithelial, dormant) and, like all osteoblasts, build and structure the extracellular matrix bone. Nor do we want to discuss the regulating influence of osteocytes on bone metabolism, but rather we will focus on the ‘bone-degrading’ cell type, the osteoclast.
In contrast to the other three cell types that belong to the lineage of osteoprogenitor cells, the osteoclast derives from the lineage of monocyte-macrophage progenitor cells from the bone marrow. In other words, monocytes are the precursor cells of osteoclasts. Monocytes are formed in the bone marrow during monocytopoiesis. Mature monocytes are distributed throughout the body by the vascular system, i.e. by blood circulation. Either they remain there (with an average residence time 12-48 hours), take up pathogens by direct phagocytosis, then migrate to the nearest lymph nodes for antigen presentation, or they migrate to peripheral tissue where they differentiate into macrophages, partly into dendritic cells or (in bone tissue) into osteoclasts.
In bone, monocytes fuse to form multinucleated cells that can contain up to 30 cell nuclei. This process, known as osteoclastogenesis, is regulated by the osteoblasts, osteocytes, and stromal cells of the bone marrow.
After the adhesion of the osteoclasts to the surface of the target bone matrix, they create a locally very limited acidic environment in a separate space between the bone surface and the cell membrane. This acidic milieu is necessary for bone resorption and initially involves the dissolution of the inorganic components of the bone (bone demineralisation), which is mediated by the enzyme H+-ATPase. After a time delay, the enzymatic degradation of the organic components follows, amongst others by the lysosomal protease Cathepsin K.
This shows that osteoclasts play a decisive role in the renewal and remodelling processes of bone. The physiological bone remodelling processes occur continuously and in many different places simultaneously. The resorbed bone matrix is replaced with a new one, a process that is managed by the osteoblasts. Chemically, this is a dynamic equilibrium between the degradation and formation of bony extracellular matrix (bECM).
Osteoclasts are relatively large cells whose diameters can measure up to 100µm. As can be seen from the previous description, they are highly polarised cells which are located between a basal pole facing the bone surface and an apical pole facing away from the bone surface. Osteoclasts generally sit with their basal cell pole on the surface of the bECM in flat concavities called ‘Howship’s Gap ‘or subosteoclastic compartment.
The cell membrane of the basal cell pole, which faces the lacuna, has many deep folds, the osteoclastic ruffled border. In a state of inactivity (resting phase) this ruffled border disappears.
At the transition between the ruffled border and the lateral non-crimped cell membrane, there is a marginal zone where bECM and osteoclast cell membrane come very close. Intracellularly, a ring of actin filaments (a component of the osteoclast cytoskeleton) concentrates, which guarantees a sealing of the bone resorption zone via cell membrane-bound integrin receptors with components of the bECM. This is referred to as the sealing zone of Howship’s bone resorption lacuna.
The cytoplasm of osteoclasts is rich in mitochondria, which in the form of adenosine triphosphate (ATP) provide the chemical energy for the hydrogen ion pumps, which in turn are responsible for the acidification of the subosteoclastic compartment and for the subsequent activation of lysosomal and non-lysosomal enzymes.
Osteoclasts can be temporarily activated to mobilise bivalent calcium ions from bone to blood. They thus react very quickly to a metabolic demand. Their activity is directly controlled by calcitonin, vitamin D3 and other molecules produced by osteoblasts and bone marrow stromal cells. Fig. 1 shows an active osteoclast sitting on the bone surface and forming a Howship’s bone resorption lacuna.
At the apical pole, bicarbonate-chloride ion exchange channels guarantee the maintenance of cytoplasmic pH neutrality. Additionally, chloride channels at the basal pole prevents an excessive increase of the intracellular pH. Within the cell, the carbonic anhydrase II ensures the production of protons from carbon dioxide and water. These protons are released into the lacuna by an ATP-dependent pump to generate an acidic pH of about 4.5 and thus dissolve the bECM. Bicarbonate is exchanged for chloride. The chloride is then released into the subosteoclastic compartment through the chloride channel. Proteinases, phosphatases (lysosomal enzymes), and metalloproteinases (non-lysosomal enzymes) are released into this compartment as well to degrade the organic components (collagen and other proteins) of the bECM.
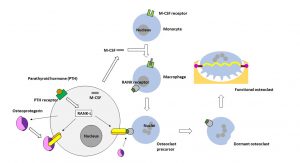
Regulation of osteoclast differentiation and activity
How complex the relationships actually are cannot be described in the context of this short article, but without osteoblasts or osteoblastic function there would be no osteoclasts. Osteoblasts are derived from pluripotent mesenchymal stem/stromal cells (the correct naming of this pluripotent cell type is remains very controversial at present) which are also the precursor cells of muscle cells (muscle tissue), adipocytes (fatty tissue), fibroblasts (connective tissue), and chondroblasts (cartilage tissue).
Osteoblasts express and secrete macrophage colony-stimulating factor (M-SCF), which is essential for the survival and proliferation of monocytes or macrophages, the precursor cells of osteoclasts (see Fig. 2). Stromal cells of the bone marrow and osteoblasts produce the ligand RANK-L with binding affinity to the receptor activator of nuclear factor kappa B (NF-κB). NF-κB is an important transcription factor heterodimer that is activated by inflammatory or other immunologic signals.
Both proteinogenic biomacromolecules M-CSF and RANK-L, which are synthesised by osteoblasts after parathyroid hormone stimulation, are required for the differentiation of monocytes into macrophages and the formation of osteoclastic precursor cells. As in all physiologically balanced equilibrium processes, there is a counter-regulation mechanism that can prevent osteoclastogenesis (the formation of osteoclasts). For this purpose, osteoblasts secrete a glycoprotein called osteoprotegerin. This glycoprotein binds to the RANK-L with a higher affinity than the RANK receptor, thus serving as a ‘decoy’ protein and preventing the completion of differentiation into functional osteoclasts.
However, when functional, bone-degrading osteoclasts are required, the parathyroid hormone blocks the synthesis of osteoprotegerin and osteoclast precursor cells (multinucleated macrophages) can bind via their cell membrane-located RANK receptor to RANK-L that is presented on the surface of osteoblasts. Once these osteoblast-osteoclast precursor contacts are established, the multinucleated macrophages turn into multinucleated immature osteoclasts. These immature osteoclastic cells separate from the osteoblasts and enter a dormant, non-degrading state on the bone surface (dormant osteoclasts).
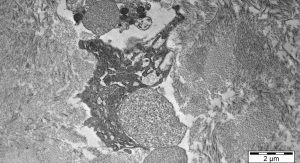
Diseases in which osteoclasts play a major role and possible therapies
Osteoporosis is a disease of the entire skeleton, in which not only the bone mass decreases, but in particular a pathological degradation of the bone structure occurs which increases fragility and thus the risk of bone fractures. At present in Germany, an estimated 5.2 million women and 1.1 million men over 50 years have osteoporosis. Every year, 885,000 people in Germany contract osteoporosis (Hadji et al, 2013). Mechanistically, this is attributed to excessive osteoclastogenesis, a pathologically increased activity of osteoclasts and a reduced synthesis of bone substance due to increased RANK-L release. Besides a basic therapy with a sufficient daily supply of calcium and vitamin D (the daily requirement is 1,000mg of calcium and 800-1,000 IU (International Units) of vitamin D), various osteoporosis medications can be prescribed. One such class of drugs are bisphosphonates.
Periprosthetic osteolysis (PPOL) is a complication that can occur after total joint arthroplasty. For example, according to current knowledge, aseptic PPOL is caused by wear particles, which ultimately lead to increased osteoclastogenesis and osteoclast activity. The therapies of choice are currently revision surgery and the administration of bisphosphonates. The latter, by inhibiting a synthetase, can inhibit the polarisation of osteoclasts and thus their activity. Other osteoclast-related diseases are rheumatoid arthritis, bone tumours, Paget’s disease, and osteopetrosis. An excellent review about these diseases, the mechanistic background and the involvement of osteoclasts and possible therapies, is given by Bi et al (2017).
References
1 Salber, J. ‘The curse and blessing of total joint arthroplasties: the only effective therapy for patients suffering from advanced osteoarthritis’. The Innovation Platform. 2020;1:166
2 Kierszenbaum, A.L. Histology and Cell Biology – An Introduction to Pathology. Mosby Inc., St. Louis, 2002
3 Hadji, P., Klein, S., Gothe, H., Häussler, B., Kless, T., Schmidt, T., Steinle, T., Verheyen, F., Linder, R. ‘The epidemiology of osteoporosis—Bone Evaluation Study (BEST): an analysis of routine health insurance data’. Dtsch Arztebl Int 2013;110(4):52–7
4 Bi, H., Chen, X., Gao, S., Yu, X., Xiao, J., Zhang, B., Liu, X., Dai, M. ‘Key Triggers of Osteoclast-Related Diseases and Available Strategies for Targeted Therapies: A Review’. Front. Med. 2017;4:234
Co-authors
Dr Mustafa Citak
Consultant Orthopedic Surgeon
Director of Research Department
Fellowship Director bei Helios
ENDO-Klinik Hamburg
+49 40 3197 0
mustafa.citak@helios-gesundheit.de
www.helios-gesundheit.de/kliniken/hamburg-endo-klinik
Dr Inge Schmitz
Cell Biologist, Electron Microscopy Specialist
Institute of Pathology
RUHR-University Bochum
+49 234 302 4703
inge.schmitz@ruhr-uni-bochum.de
Dierk Gruhn (B.Sc. Biochemistry)
Master’s student
Department of Experimental Surgery
dierk.gruhn@rub.de
Lead author
Dr Jochen Salber
Senior Physician, Surgery
Head of the Department of Experimental Surgery
Department of Surgery
University Medical Centre Knappschaftskrankenhaus Bochum
Hospital of the RUHR-University Bochum
+49 234 32 21819
jochen.salber@rub.de
www.kk-bochum.de
Please note, this article will also appear in the fourth edition of our new quarterly publication.