Phage therapy emerges as a promising solution against antimicrobial resistance by utilising highly selective viruses that target specific bacteria, though its effective deployment faces challenges related to matching phages with infections and regulatory considerations.
Antimicrobial resistance (AMR) poses a serious threat to our ability to treat bacterial infections and is now one of the top global threats to public health and economic development. Combatting AMR requires action in many areas to address the interrelatedness of human, animal and environmental health, known as the One Health approach.
Background
In 2024, the World Health Organization (WHO) provided a grim warning that “not only are there too few antibacterials in the pipeline, given how long is needed for R&D and the likelihood of failure, there is also not enough innovation.” The WHO also reviewed the newly approved antibacterials which had been released since 2017. Of the 13 newly approved antibiotics gaining authorisation, only two represent a new chemical class. This apparent lack of innovation illustrates both the lack of big pharma focus in this space and the scientific and technical challenges to discovering novel antibacterials that are both effective against bacteria and safe for human use.¹
With a scarcity of new and innovative antibiotics becoming available and the rapidly increasing occurrence of AMR, attention is turning to bacteriophages (phages) as a promising weapon against AMR.
Phage therapy as a weapon to combat AMR
Phages are viruses that have evolved to be highly selective in infecting bacteria, frequently infecting only a small subset of a particular bacterial strain, which in turn is a subset of a bacterial species, e.g. E. coli. This specificity for very specific limited set of bacteria is concomitantly one of phage therapy’s greatest benefits and one of the biggest challenges to overcome. Such specificity enables phage to remove only harmful bacteria, leaving other, beneficial bacteria unaffected. However, this means that phage therapies need to be carefully matched to the specific bacteria causing the infection and/or the use of cocktails containing multiple different phages, each showing specificity to the target bacteria.
Phages are the most abundant organisms on Earth and are found in every natural environment. Phages play crucial roles in regulating bacterial populations and influencing microbial ecosystems. It is estimated that there are 1031 phages in the world, equivalent to 1 trillion phages in existence for every grain of sand², which would amount to a biomass of 200 million tonnes.³ The result is that that each bacteria will have multiple phages capable of targeting it which, on one hand, means that there will be a phage in the world to tackle every bacterial infection but, on the other hand, it can take a lot of hunting to find the right one(s).
Broadly, phages come in two types: lytic and lysogenic. Lytic phages follow the lifecycle as illustrated in Fig. 1, quickly replicating and bursting out of the cell, resulting in the death of the bacterial cell. In contrast, lysogenic phages do not immediately replicate. Instead, their genetic material is inserted into the host cell’s genome, creating what is known as a prophage. These prophages are replicated as the host cell proliferates but can be triggered to become lytic. For therapeutic purposes, it is important that only lytic phages are selected and that any phages with lysogenic potential are excluded.
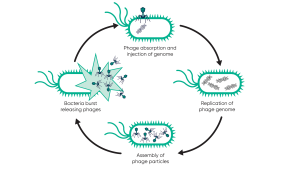
Phage – the potential and the challenges
The abundance and variety of phages, coupled with the co-evolutionary nature of both phages and bacteria, presents a unique challenge to deployment and the regulation of this class of therapy. Commonly, one of two options are available when considering phage therapy: 1) non-personalised phage cocktails containing pre-formulated phages against common infections, or 2) personalised phage therapy made in response to the individual’s specific infection.
In a recent review of 100 human phage therapy treatments, the use of personalised phage therapy was more effective than non-personalised, especially when used in concert with antibiotics.⁴ In March 2025, a team at Singapore General Hospital (SGH) successfully treated a patient with a persistent Pseudomonas aeruginosa infection following cardiac surgery, which could not be resolved by antibiotics. Following repeated hospitalisations, the patient received a two-week course of phage therapy, which treated the infection effectively.⁵ Despite being highly effective, this approach is not currently practical for mass use as the effective phage-host combination needs to be tailored to the individual infection.
In addition to human health, phages have applications in other industries, from veterinary medicine to hygiene and sanitation (Fig. 2). In these areas, the more common approach is for organisations to prepare a cocktail of multiple phages to provide broader efficacy against a target strain or strains. One such example is the ‘BAFASAL + G®’ poultry feed additive produced by Proteon Pharmaceuticals S.A., which consists of six phages targeting different variants of Salmonella, which improves yield and food safety whilst preserving a healthy chicken microbiome.⁶

Phage therapies and treatments offer great promise to improve human, animal and crop health. However, widespread adoption of phage therapy is not yet a reality and requires input from numerous stakeholders across the industries, from regulatory bodies to biopharmaceutical companies and technology providers. The unique and complex nature of the phage-host interaction, other biological factors, and societal factors all present challenges, outlined in Fig. 3.
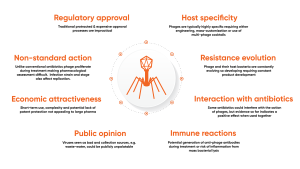
Despite these challenges, the industry is witnessing a significant increase in the number of successful use-cases within clinical settings, the prophylactic use of phage in agricultural settings and the development of phage-impregnated sanitary materials. This increasing interest in phage-based technologies is reflected in increasing production requirements and process controls, which necessitate a transition from small-scale, flask-based culture to a bioreactor-based approach.
Increasing the production scale presents challenges as phage particles are pervasive and can be very difficult to remove from surfaces. The persistence of phage requires bioprocess scientists to employ complex and costly molecular biology-based methods to validate cleaning processes to prevent cross-contamination between batches. In addition to complex cleaning validation, re-usable bioreactors require lengthy and energy intensive de-commissioning/re-commissioning cycles between production batches. Such delays and costs are incongruous with the needs of phage therapy production, which needs to be quick to react to immediate needs and be as cost-effective as possible.
To this end, the Cellexus’ CellMaker bioreactor is rapidly becoming established as the bioreactor of choice for organisations looking to scale up phage production.
CellMaker: The go-to bioreactor for phage production
CellMaker enables phage to be produced in higher quantities, in less time and at lower cost than previously possible by;
Eliminating cleaning
CellMaker employs single-use bioreactor bags, removing the need for costly cleaning and validation steps that are required when using conventional bioreactors. Manufactured to ISO13485 & FDA 21 CFR 820 processes with regulatory support available
Maximising system up-time
By removing the need for lengthy and costly cleaning, decommissioning and recommissioning steps, a CellMaker bioreactor can be ready to go again within a matter of minutes. Simply put in a new bag, fill with media and the system is ready for use. Practically negating downtime a facility can maximise production capacity, increase team productivity and always be ready to respond to need.
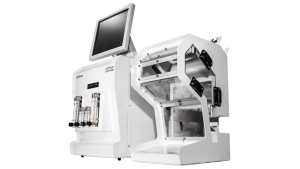
Being able to rapidly change culture volumes
CellMaker systems are ‘plug and play’, quickly exchange enclosures to scale from 1.5-litre to 50-litre culture volumes using the same CellMaker Controller. Dual Controllers expand this further by being able to independently control two enclosures, providing production capabilities from 1.5 litres to 100 litres with a single controller
Increasing phage yield
CellMaker utilises a curtain of fine bubbles to effect both mixing and gas exchange, known as Airlift mixing, which has been shown to produce significantly higher (10-100x) titres of phage than other methods. Higher concentrations enable the use of smaller culture volumes, further reducing production time and costs and positively impacting downstream processing.
Versatility
Being a closed, single-use system that is capable of culturing a wide range of cell types in aerobic or anaerobic conditions, CellMaker can be used to produce a variety of products or to use in different culture processes.
Cellexus is committed to supporting phage scientists and developing solutions to meet evolving needs across sectors and geographies. Contact us if you would like to discuss how we could help your workflow.
References
- WHO website
- Browning, D. (2025). Bacteriophage: Translation into clinical practice. Industrial Pharmacy 74, 6-10.
- Chevallereau, A., Pons, B.J., van Houte, S. et al. (2022). Interactions between bacterial and phage communities in natural environments. Nat Rev Microbiol 20, 49–62. https://doi.org/10.1038/s41579-021-00602-y
- Pirnay, JP., Djebara, S., Steurs, G. et al. Personalised bacteriophage therapy outcomes for 100 consecutive cases: a multicentre, multinational, retrospective observational study. Nat Microbiol 9, 1434–1453 (2024).
- The Straits Times
- Proteon Pharmaceuticals S.A. website
Please note, this article will also appear in the 22nd edition of our quarterly publication.