Dr Michèle Levi spoke to International Editor Clifford Holt about how she plans to use her Ernest Rutherford Fellowship to develop our fundamental understanding of gravity from the smallest quantum to the largest cosmological scales.
Dr Michèle Levi has recently received a prestigious Ernest Rutherford Fellowship from the Science and Technology Facilities Council (STFC). She plans to use this to develop a programme to deliver analytical high-precision predictions for real-world gravitational-wave data and to develop our fundamental understanding of gravity from the smallest quantum to the largest cosmological scales. The programme will address the high demand for accurate theoretical templates of gravitational waveforms from two merging compact objects, such as black holes.
Pushing the precision frontier for gravitational waves is one of the most urgent tasks in theoretical physics today, in light of the increasing influx of data from a rapidly growing worldwide network of gravitational-wave detectors. Levi will analytically predict gravitational radiation from such compact binaries and further develop the use of quantum field theory advances to study gravity. She seeks to uncover profound duality relations between gauge and gravity.
International Editor Clifford Holt spoke to Levi about her research, the importance of analytical high-precision predictions for real world gravitational wave data, and potential challenges as she works to develop a better idea of universal commonalities across classical and quantum field theories.
Firstly, congratulations on being awarded the Ernest Rutherford Fellowship. How important are funding mechanisms such as these, and what will the Fellowship mean for your own research and career?
Thank you! A funding mechanism like this prestigious early-career award from the STFC (one of the seven UK research councils under UKRI) is very important and unique. This is the most competitive scheme in the UK for early-career researchers in my domain, devised to ensure that those 10 carefully-selected awardees, who are recognised as the very top international talent and leadership ‘material’ in science early on in their career, will be integrated into top academic positions in the leading research institutions in the UK.
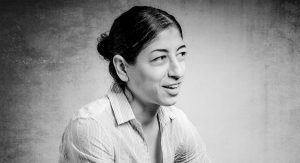
This will enable the awardees to play a key role in the coming decades in the scientific and transformative leadership at the national level in the UK and will also represent the UK at the international level, solidifying its position as a scientific world-leader for the next generations. As a public funder, UKRI selects these future leaders to shape strategic UK policies, to bolster the UK’s scientific place in the world, and to bring about a radical shift in the current research culture in terms of the way that science is conducted, namely its standards and its values, but also its role and commitment within society.
Currently, many academics have sadly forgotten that their raison d’etre is to serve the public, that they have the privilege of expanding the frontiers of knowledge mainly thanks to the public money that is funding their research. We must insist that science that is peer-reviewed and approved for publication and funding lives up to the standards of FAIR (Findabilty, Accessibilty, Interoperabilty, Reusabilty) principles, namely of responsible research of integrity, that is publicly available, widely accessible, and reproducible. We should also advocate for equality, diversity, and inclusion in Science, which should properly represent Society, and engage with the public. It is very clear that UKRI as a public funder has a strong commitment to realising these aspirations, which are unfortunately still overlooked by the majority of private funders.
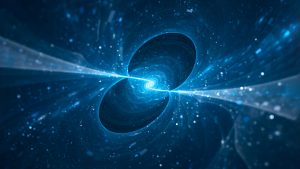
I am excited and honoured to transfer and base my cutting-edge research programme in the UK, and to be part of making the UK a world-leading force in the timely domain of gravitational waves with the most innovative theoretical approaches and computational tools applied to gravity. I am also incredibly aligned with the aspirations of UKRI to transform the current research culture, and as I myself belong to an overlap of under-represented and marginalised groups in science, I am particularly aware to how systemic inequalities affect the ability of students and researchers to truly realise their potential and excel, or even to just conform to the common skewed metrics of academic merit.
I adamantly champion and pursue equality, diversity, and inclusion in every aspect of my everyday scientific activities. I believe and I see that it is only through these consistent everyday actions and through my being a role model that I can ultimately translate my research work to further concrete scientific and societal impact. I also adamantly advance the broad communication of science across the research community and through public outreach, which I regard as a social imperative. I also find it extremely rewarding to share my knowledge with as many people as possible.
Your project aims to deliver analytical high-precision predictions for real world gravitational wave data. Can you tell me more about this and why it is important?
Gravitational radiation is a probing prediction of any complete theory of gravity. Currently, we still do not have a complete theory of gravity and this is true for both ends of the scale, since we do not know how to describe gravity on quantum scales, which are the smallest scales, and we do not have a theory that captures gravity on the largest scales – cosmological scales, since we do not know how to explain the puzzle of dark matter or the accelerated expansion of the Universe that was discovered in 1998. Understanding how the force of gravity works on these more ‘extreme’ scales is possibly the deepest and most fundamental longstanding open problem in physics.
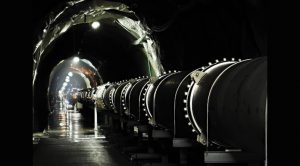
The first Earth-shaking gravitational-wave (GW) detection announced in 2016 launched a new era of gravitational-wave astronomy and precision gravity, which is only in its infancy, with vast future impacts in astrophysics and cosmology and, most notably, in fundamental physics. Since this first milestone detection, ground-based detectors keep multiplying, and many more are planned for the coming years. They are designed to measure various frequency ranges, which will enable a richer array of sources and events that generate gravitational radiation to be captured.
The technology of measurements is constantly upgraded, either in currently-operating detectors like LIGO or VIRGO, or in upcoming and planned ones, like KAGRA in Japan, and others in the US, Europe, India, and Korea. Furthermore, there are also concrete planned space-based experiments from Europe, China, and Japan that will also target smaller frequencies and other types of sources. All in all, in the coming years and decades we are headed towards a growing influx of gravitational-wave data that will have higher and higher quality.
Let me stress what, I believe, much of the community in Europe and the US does not fully recognise yet: that Asia is about to emerge as possibly the major player in gravitational-wave astronomy in the coming years: in Japan, KAGRA is already operational; in India, IndiGO will become operational in just a few years; and in Korea, SOGRO is planned to uniquely target mid-range frequencies from Earth, while China and Japan are also planning space-borne detectors, TianQin and DECIGO, respectively.
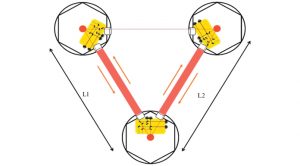
How will your research help to inform the activities of detectors such as VIRGO and LIGO, as well as perhaps LISA? How could your theoretical work come to complement future observations/detections?
My research generates theoretical data from which a huge bank of templates is created to be matched in the detectors by the real data that is measured. The GW signal is weak and is covered by many sources of noise so, in order to identify it the matched filtering technique is used for detection, which requires the most accurate theoretical signal templates possible. When a match is found, a detection is announced. Yet, lots of real-world data, even if is great data is just data without interpretation. What transforms data into knowledge is theory and analysis. This is especially true for the gravitational-wave signal since it is so weak, so it is really important to separate the noise from the true signal.
For the most part, the observable differences among the plethora of candidate theories for gravity suggested along the years have been insanely tiny. In most cases, all of these theories seem grossly similar within gravitational-wave measurements. So it is only the theoretical resolution into various physical effects at a very high-precision, such as those that have been computed in my research since my graduate studies, that will enable us to really distinguish among the various suggested theories of gravity, and to close in on which is the viable one.
Another feature which is rather unique to my own theoretical framework is that it provides predictions for generic sources of compact binaries, not only for binaries of two black holes. As the technology in upcoming detectors advances, and as the sensitivity improves, we are able to observe more and more neutron stars, for example, in the radiating binaries. Whereas from black holes we can ‘only’ learn about gravity theories, neutron stars also tell us about the theory of QCD (Quantum Chromodynamics) in extreme conditions that no human-built lab could produce. With future detectors, we also expect to observe white dwarfs, and learn more about them.
Have you yet been able to identify any potential challenges that you expect to have to overcome as you work to develop a better idea of universal commonalities across classical and quantum field theories?
Right now, we see some analogies between interactions of classical extended gravitating objects like the huge non-rotating black holes in radiating binaries, and elementary particles of quantum nature, which we call ‘scalar particles’ – these are boson particles of zero quantum spin. But the precise mapping between them, and more importantly its generalisations for all types of elementary particle, is poorly understood. In particular, my theoretical work pushed the understanding of spinning gravitating objects, which would also be analogous to higher-spin particles. Currently, the highest-spin particle that has been observed in nature is of spin one, and we also believe there could be particles of spin three/two according to supersymmetric theories, as well as our theoretically predicted graviton – a massless particle of spin two. But what about particles with spins larger than two? Do they exist? Could they be elementary? Or only show up as what we call ‘composite’ particles, which must be composed of elementary ones?
At the moment, we cannot even manage to give well-defined predictions of observables of quantum field theories that capture such higher-spin particles, and we are certainly not able to write down such quantum field theories explicitly. Furthermore, we are not certain whether this fundamental issue exists already at the classical level, i.e. with the type of two-body interactions that my work is tackling for spinning gravitating objects. The big challenge for my research programme, therefore, would be to more rigorously and generally link the classical and quantum sides of these analogies and to clarify whether there is indeed a fundamental issue with higher-spin – and, if so, whether it originates in the quantisation of the theory, or if it already exists at the classical level.
This all relates to possibly the biggest open question in theoretical physics of how to complete the theory of gravity at high energies, and therefore it would indeed be a breakthrough for my upcoming research programme to be able to provide any further insights on that.
What are your short/long term hopes and ambitions?
Since my graduate studies, I have had the privilege of pioneering and leading innovative analytical work and high-precision computation on the highly intricate problem of compact binaries that source gravitational radiation. At the time, I did not believe that I would live to see actual detections of gravitational waves from these sources, since at that time the detectors had been operational for more than a decade without any successful detection.
Yet, the detection came in February 2016, and I can still remember it as though it were yesterday; I was overwhelmed with excitement and wonder for what was to come when the first observation of a gravitational-wave signal by LIGO was announced that day. Since then, the scientific prospects of gravitational-wave science have been continuously improving and exceeding all expectations. The future truly looks symphonic, filled with gravitational-wave echoes.
With this increasing influx of data of an ever improving quality, we have a chance to really push theoretical predictions and to test so much more theory in gravity and even in QCD. My research programme is exactly aimed at maximising the potential of what we can learn from gravitational-wave measurements on the theory of gravity across all scales.
I hope to engage the broader scientific community with this exciting new domain of research, such that it really crosses over all sub-fields of theoretical physics, especially since this is a great scientific challenge that can benefit from so many different specific perspectives. I believe that every one of these perspectives can bring in something fresh and interesting to the mix. I also believe that, to some extent, I have already contributed to the widespread attention that this type of research obtained in the traditional high energy physics community. In the UK, I hope to engage more people and institutions with the research in this field as the UK really has the potential to become a superpower in this domain, especially in the type of analytical work that I am involved in, which has been traditionally dominated by France.
I am also a big believer in open code and open data, and I really want to see individual researchers, big experimental collaborations, scientific journals, and academic institutions all persistently moving towards this in the coming years. The scientific challenges at hand are too big, and we need to focus on the science and make our best joint efforts to make breakthroughs, instead of yielding to the old zero-sum game mentality that keeps theoretical physics stagnated.
Simply put, I just hope that, together, we can all increase our knowledge of the world around us. Isn’t that the greatest privilege of our society?
Dr Michèle Levi
https://sites.google.com/view/levim
Please note, this article will also appear in the seventh edition of our quarterly publication.