Members of the National Agriculture and Food Research Organization, Japan, have been conducting ‘Free-Air CO2 Enrichment’ experiments to ascertain the impact that climate change is having on rice production.
Rice directly feeds more people than any other crop and is relied on as a staple food source by half of the world’s population. But while its adaptability allows it to grow in a wide range of conditions, from cold to tropical climates and low to high altitude areas, projected climate change is becoming a major threat to the stable production of high-quality products.
Atmospheric CO2 concentrations are rising at an unprecedented rate, along with other greenhouse gases, and we are seeing increasing temperatures and differing precipitation amounts and patterns, as well as more frequent occurrences of extreme weather.
But, while many of these changes are projected to have negative effects, an increase in CO2 has a positive effect on rice crops – enhancing photosynthesis, biomass, and thereby grain yield – through a phenomenon known as ‘CO2 fertilisation’.
Early studies used enclosure chambers to determine the crop’s response to increases in CO2 but there was a growing need to test CO2 fertilisation in the open field and in 1998, a technology known as ‘Free-Air CO2 Enrichment’ (FACE) was applied to a paddy field in Japan (see Figs 1 and 2) by members of the National Agriculture and Food Research Organization, a Japanese research facility headquartered in Tsukuba Science City, Ibaraki.
Since then, they have conducted a series of FACE experiments on paddy fields in Shizukuishi in northern Japan, and Tsukuba in central Japan, to find out how rice crops grown in cold and warm climates respond to high CO2 in order to design crop cultivar and management protocols for the future.
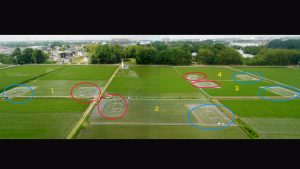
Do yield responses to elevated CO2 differ between warm and cool FACE sites?
FACE experiments have been carried out, using a common cultivar, at the two sites over 13 seasons and have shown that yield increased by 11% on average in response to elevated CO2 (+200ppm above the current level) but the effect varied considerably (0-21%) from year to year.2
The experiments have shown a correlation between decreasing grain yield enhancements and rising temperatures, with yield enhancements falling by 2.4% for every degree that the temperature goes up (see Fig 3). This suggests that the positive effect of elevated CO2 on yield may be partially offset in future warmer climates.
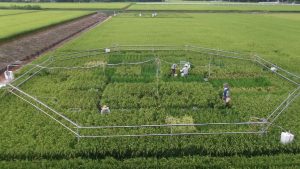
Do yield responses to elevated CO2 differ between rice producers?
Yield enhancements due to elevated CO2 differed largely between cultivars ranging from 3 to 36%, suggesting that great opportunities exist for improving rice productivity in the future and higher yielding cultivars with large panicles or large grains showed a greater CO2 response.3-6
In rice, some genes that confer greater grain numbers have been identified7, 8 and the research has shown that introducing these genetic regions into conventional cultivars can improve grain yields in elevated CO2.9 This strategy can be used to develop high-yielding varieties under elevated CO2.
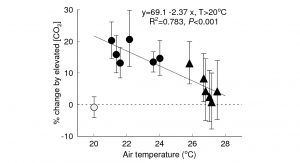
Does elevated atmospheric CO2 degrade grain quality in rice?
Grain quality is becoming increasingly important in the rice market, and serious concerns are emerging about quality losses due to heat stresses under global warming.10, 11 The amount of chalky immature grains increases with rising temperatures during the grain growth stage and this decreases marketability and milling quality. The FACE experiments have shown that elevated CO2 also significantly degrades the grain appearance quality by increasing the percentage of chalky grains12, which is particularly pronounced in hot years.13
Simultaneous increases in CO2 and air temperature will negatively impact high-quality rice production, while elevated CO2 also decreases zinc, iron, and protein content in the grains.14, 15 It is important to note that considerable variation exists in the quality degradation across different rice cultivars,14, 15 which suggests that there is scope for breeding rice cultivars whose micronutrient levels or appearance quality are less vulnerable to increasing CO2.
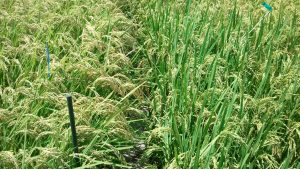
Do CO2 and varieties matter in micro-, local-, and global climate systems?
The expected adverse effects of global warming on crop production are alarming, but the size of the impact is not solely dependent on the air temperature. Climatic and physiological factors that affect tissue or sensing organ temperatures can induce heat stress.
Elevated CO2 increases daytime canopy temperatures by as much as 1°C through its influence on stomatal conductance,16 which could, in turn, exacerbate heat stress under hot conditions. Canopy cooling by transpiration could potentially alleviate heat stress, but this needs to happen at the expense of greater water use.
Two cultivars with contrasting transpiration capacity have been studied during the experiments. Takanari, a high-yielding indica cultivar, was identified as a candidate for high productivity under elevated CO2 (see Fig 4) and for comparison, researchers chose a japonica cultivar Koshihikari, the main rice cultivar grown in Japan for over 50 years.
At a single-leaf level, Takanari had 30 to 40% higher stomatal conductance (a measure of stomatal opening), but at the canopy level, the difference in evaporation reduced to 4-5%, which could be cancelled by decreased evapotranspiration by elevated CO2. Despite the small difference in evapotranspiration between these cultivars, the model simulations showed that ‘Takanari’ clearly decreased canopy and air temperatures within the planetary boundary layer compared to ‘Koshihikari.’ This indicates that lowland rice varieties characterised by their high stomatal conductance can play a key role in enhancing productivity and moderating heat-induced damage in response to climate change without significantly increasing crop water use.17
Additionally, Takanari’s canopy architecture with erect leaves may also contribute to lower canopy temperature, which could also have an impact on micro and local climates.18
Compared to other major cereals, rice is peculiar as it is mostly grown under submerged conditions with irrigated and rain-fed lowland areas accounting for more than 80% of the rice-growing regions.1
Rice paddy accounts for about 11% of global methane (CH4) emissions19. FACE experiments combined with soil warming by 2°C indicated an increase in CH4 emission by as much as 80%, suggesting that climate change promotes CH4 emissions from paddy fields, which in turn accelerates global warming.20
There is a strong need to quantify these effects to mitigate greenhouse gas emission in paddy fields effectively and because much of the CH4 emitted to the atmosphere is through plants21, understanding plant responses and carbon flow under climate change is the key to estimate and control CH4 emission from paddy fields. FACE was also used for a large open field carbon tracer experiment to quantify the source of carbon for CH4 emission from the paddy field and found that about half of CH4 released during the growing period originated from the current season photosynthate.22 This finding highlights the need to understand carbon flow in the soil-plant-atmospheric continuum quantitatively.
References
- GRiSP (Global Rice Science Partnership), Rice Almanac. (International Rice Research Institute, Los Banos, Philippines, 2013), pp. 283
- Hasegawa et al., in Improving Modelling Tools to Assess Climate Change Effects on Crop Response, J. L. Hatfield, D. Fleisher, Eds. (American Society of Agronomy, Madison, WI USA, 2016), pp. 45-68
- Hasegawa et al., in Functional Plant Biology. (2013), vol. 40, pp. 148
- Hasegawa et al., in Frontiers in Plant Science. (2019), vol. 10, pp. 1-15
- Sakai, T. Tokida, Y. Usui, H. Nakamura, T. Hasegawa, in Plant Production Science. (Taylor & Francis, 2019), vol. 22, pp. 352-366
- Yoshinaga et al., in Plant Production Science. (Taylor & Francis, 2020), vol. 23, pp. 19-27
- Ashikari, in Science. (2005), vol. 309, pp. 741-745
- Terao, K. Nagata, K. Morino, T. Hirose, in Theoretical and Applied Genetics. (2010), vol. 120, pp. 875-893
- Nakano et al., in Scientific Reports. (Springer US, 2017), vol. 7, pp. 1827
- Krishnan, B. Ramakrishnan, K. R. Reddy, V. R. Reddy, in Advances in Agronomy. (Elsevier Inc., 2011), vol. 111, pp. 87-206
- B. Lyman, K. S. V. Jagadish, L. L. Nalley, B. L. Dixon, T. Siebenmorgen, in PLoS ONE. (Public Library of Science, 2013), vol. 8, pp. e72157
- Usui et al., in Rice. (2014), vol. 7
- Usui et al., in Global Change Biology. (2016), vol. 22, pp. 1256-1270
- S. Myers et al., in Nature. (2014), vol. 510, pp. 139-142
- W. Zhu et al., ‘Carbon dioxide (CO2) levels this century will alter the protein, micronutrients, and vitamin content of rice grains with potential health consequences for the poorest rice-dependent countries’. in Science Advances 4, (2018), eaaq1012
- Yoshimoto, H. Oue, K. Kobayashi, in Agricultural and Forest Meteorology. (2005), vol. 133, pp. 226-246
- Ikawa et al., in Global Change Biology. (2018), vol. 24, pp. 1321-1341
- Sikma et al., in Journal of Geophysical Research: Biogeosciences. (2020), vol. 125, pp. 1-12
- Smith et al., in Climate Change 2014: Mitigation of Climate Change. Contribution of Working Group III to the Fifth Assessment Report of the Intergovernmental Panel on Climate Change, O. Edenhofer et al., Eds. (Cambridge University Press, Cambridge, United Kingdom and New York, NY, USA., 2014).
- Tokida et al., in Biogeosciences. (Copernicus GmbH, 2010), vol. 7, pp. 2639-2653
- Nouchi, S. Mariko, K. Aoki, in Plant physiology. (1990), vol. 94, pp. 59-66
- Tokida et al., in Global Change Biology. (2011), vol. 17, pp. 3327-3337
Toshihiro Hasegawa
Group Leader
Agricultural Meteorology Group
Tohoku Agricultural Research Center
National Agricultural and Food Research Organization (NARO)
thase@affrc.go.jp
Please note, this article will also appear in the third edition of our new quarterly publication.