Richard Foster and Daniele Fregonese of Advanced Energy Minerals, alongside Frederic Falise of Scientific Visual SA, discuss the sustainability of sapphire – an important material in the watch industry.
Every time you look at your watch, you are taking advantage of the properties of sapphire without noticing them. Sapphire is important in our everyday life because of its transparency and imperviousness to scratches. In addition to watch covers, it is used in large volumes for applications from LED substrates to scratchproof windows for military vehicles.
A sizeable quantity of sapphire is used to make jewellery and watches, where high-end consumers are growing conscious of the sustainability of the products they buy. They would rather buy well-made products that last and maintain their value than cheaper goods they would have to throw away after a short time.
Industrially produced synthetic sapphire is as hard as natural sapphire but more transparent because it lacks the trace elements that give gems their various hues. Sapphire is often marketed as ‘scratch resistant’ or ‘virtually scratchproof’. This is because it measures nine on the Mohs scale of hardness, meaning that it can only be scratched by a harder substance such as diamond, which is rated at ten.1
The industrial process to manufacture and customise sapphire takes place in three major steps. The first step is to manufacture the high-purity alumina (aluminium oxide, called HPA). The second step is to crystallise this HPA at very high temperatures, of around 2,050°C, to form a raw crystal of synthetic sapphire. In the third step, this crystal mass is shaped and sliced into pieces with diamond-coated saws. The resulting wafers are then ground and polished for the desired application.
These processes cause a substantial carbon footprint that we may not think about until we are made aware of it. For instance, the total crystallisation step (second step) generates 43Kg CO2 per kg of sapphire produced. However, it can be reduced substantially by careful attention to each step.
Producing HPA
Manufacturing HPA starts with a mineral feedstock. If this feedstock is manufactured only with hydropower, its only carbon footprint at the gate of the factory where HPA is manufactured is from the transportation.
If the source is close to the factory, this can be minimal or even brought to zero by compensation. This is the case for Advanced Energy Minerals (AEM) which manufactures HPA in Québec, Canada. It uses feedstock from Québec that is produced predominantly with hydropower.
The AEM factory was designed to minimise power consumption by using a patented chlorine leach crystallisation process. Local renewable sources, hydro and wind from Hydro Quebec, provide AEM’s power. The factory at Cap-Chat is the neighbour of Le Nordais wind park with 133 turbines. Currently, renewables are at 96%, with specific plans to reach 100% by the end of the year. This will reduce CO2 emissions from the current value of less than two tonnes of CO2 per tonne of HPA to practically zero.
This is in strong contrast to those using the traditional alkoxide process, typically non-renewably powered, which emits 12.3 tonnes of CO2 per tonne of HPA. Other producers have a goal of reducing this by a factor of five, which is still very high.
Customisation to meet customer requirements makes the following process steps more efficient. AEM has a product line to deliver customised 4N and 5N compacted alumina to its customers. This ranges in size from pucks of a few grams to giant pucks of up to 21kg.
HPA to sapphire
The processes to convert HPA to sapphire all melt the alumina at a temperature above 2,050°C. This is followed by a slow cooling process that takes one to two weeks to create a crystal of sapphire. Because this is a low-tech process, the crystal is not perfect. It can, however, be influenced by the quality of the HPA used and the dexterity of the furnace operator. Lower-purity HPA is used for LEDs. The next level of purity finds application in watch covers. The highest quality HPA is transparent to deep UV due to its low titanium content. This makes it suitable for medical applications. Crystallisation issues may also impact the final product quality and determine the final application. For instance, an LED device containing an imperfect and inhomogenous sapphire component may experience decreased efficiency, a shorter lifespan, or a variation in emission colour.3
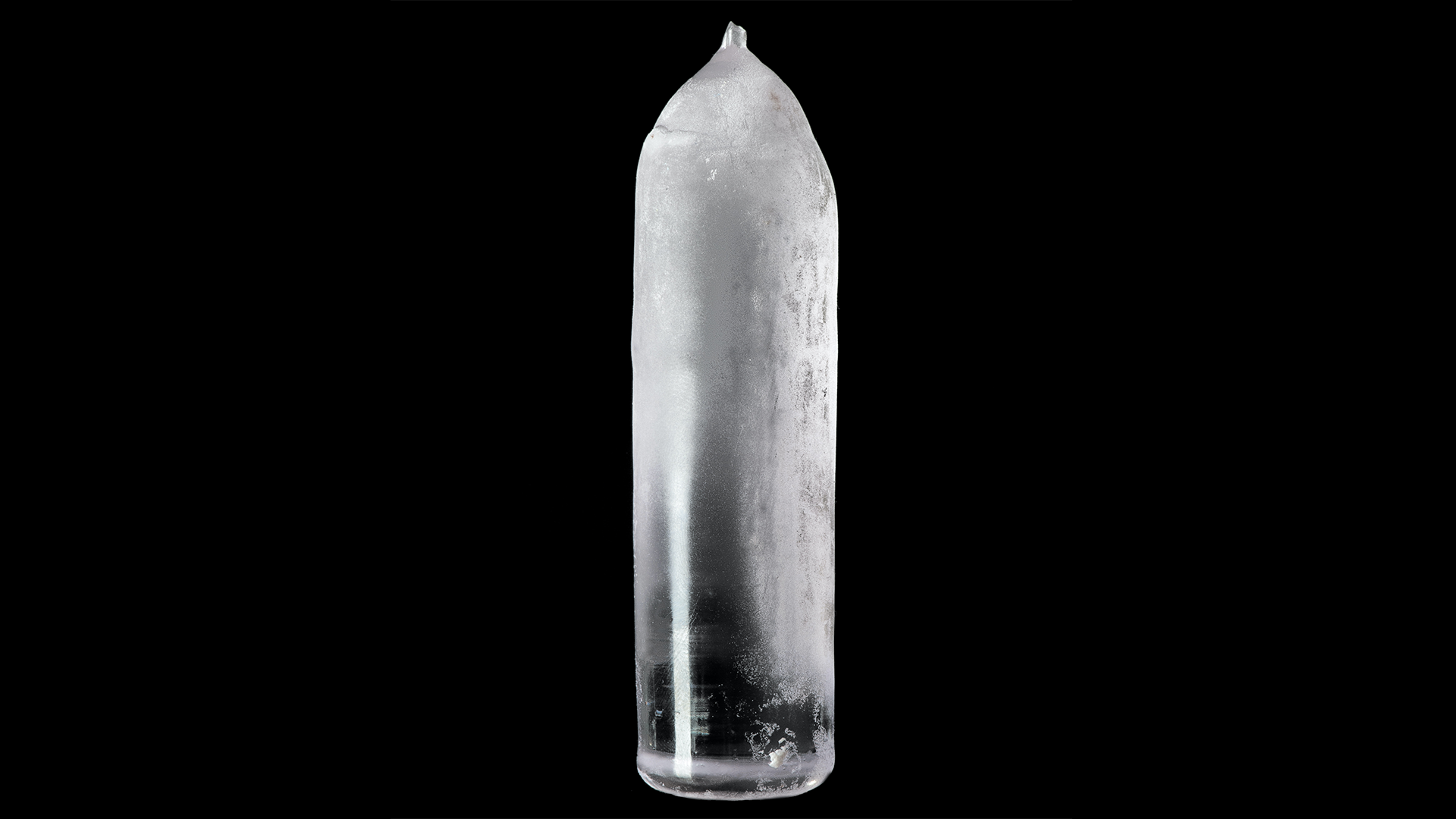
In addition to HPA quality, the different sapphire growing processes yield crystals that are suitable for different applications. For these reasons, the processes divide here. We have chosen a case study from the Swiss watch industry that shows the environmental benefits of attention to detail. The worldwide watch market of $71bn in 2022 has an expected compound annual growth rate (CAGR) of 5%.4 In particular, it is an important economic driver in Switzerland, where watch exports total almost $25bn.5 To fulfil this demand, 21 million watch covers are manufactured each year to high-quality standards in Switzerland. (There is also a huge market for smart watches manufactured in Asia that have crystal sapphire covers.) As we shall see, the remaining processing multiplies the environmental costs of the previous steps.
Sapphire crystals for watch covers are traditionally grown with the Verneuil process. The result that awaits further processing is called a ‘carrot’ in the trade. It is close to a cylinder in form, approximately 15cm high and 3-4cm in diameter.
Of course, this step has a typical energy requirement if not performed with green energy. The relevant CO2 emission would be an average of 89g/kWh for Switzerland or 475g/kWh worldwide.
Sapphire to watch cover
Because the crystal sapphire carrot has an imperfect form and contains defects, there will be substantial yield loss during the further processing steps. This loss can be minimised by generating a digital model of the carrot that is based on a 3D scan with specialised confocal tomography equipment.
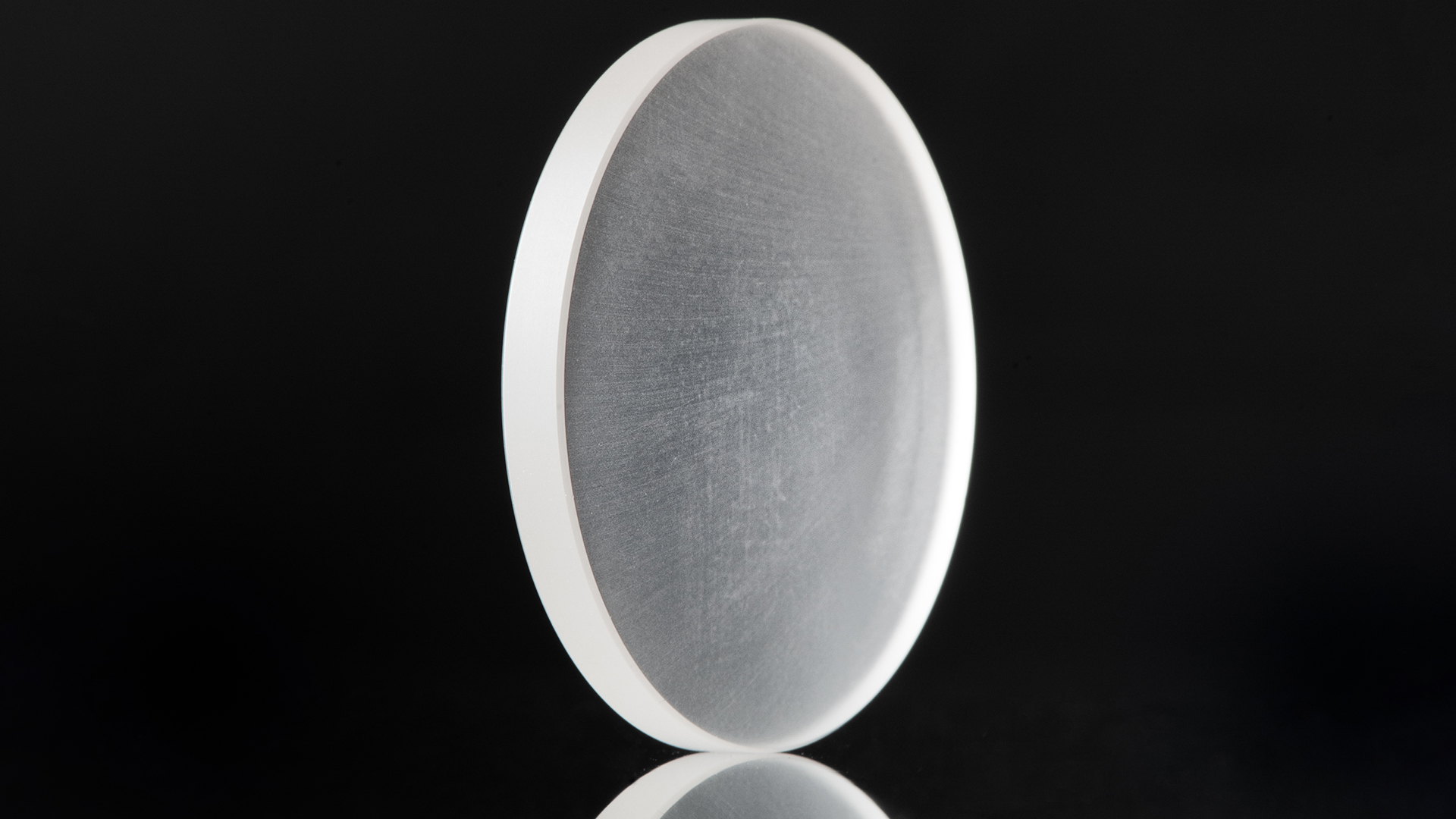
It is worth noting that cutting and polishing sapphire is highly energy-consuming, as the material’s exceptional hardness presents a significant challenge.
The first step with yield loss is the scrapping of carrots after inspection and before further processing due to an unacceptable rate of defects. This has typically averaged 10% for carrots from the Verneuil process. Grinding the remaining carrots to the correct core size and shape produces a form factor yield of 42%. The yield of cores is then 87%, meaning that 13% of cores that reach this point are thrown away because of small defects in the crystal sapphire. The yield from slicing the core into wafers and polishing them is 85%. The result is an average combined yield of 28% in the watch industry for a flat watch cover.6 This will have a diameter ranging from 25-45mm and a thickness from 1.6-6mm. The typical weight is 4.6g.
The yield means dividing the incoming environmental cost by 0.28, which almost quadruples it. For a domed watch cover, this yield is much lower at 4%, multiplying the incoming cost by 25.
These steps can all be optimised with digital quality control from the Swiss company Scientific Visual. The company manufactures scanners for quality inspection of industrial crystals (Fig. 3). Using these automated tools, individual steps can be optimised to avoid defects. For example, it prevents defective crystal parts from entering costly processing. In addition, by providing feedback to crystal growers, fewer crystals will have to be scrapped.
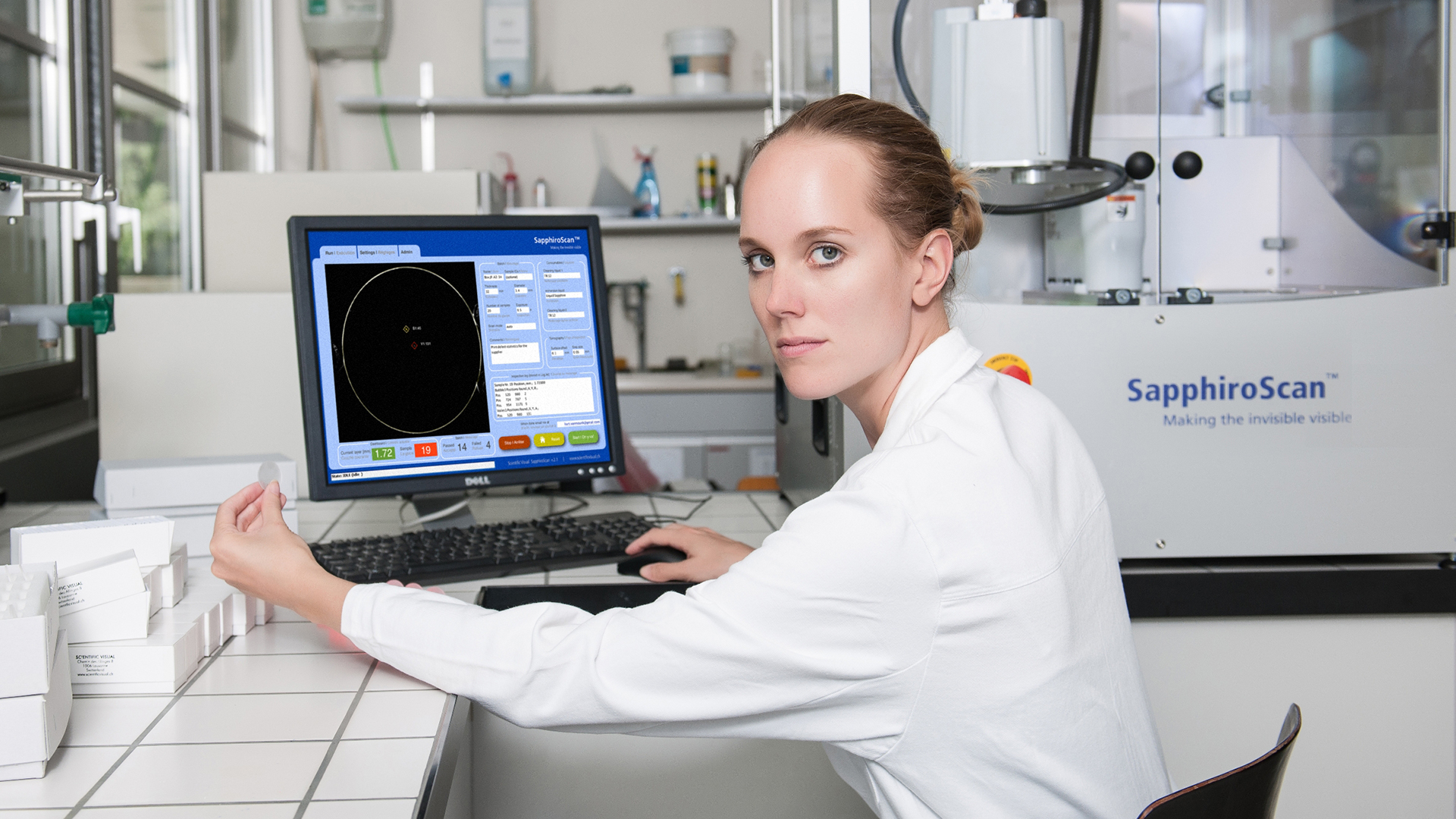
The simulated form for the cylinder can be moved so that a minimum of defects remain in the physical carrot. Slicing can also be offset to bring defects between adjacent wafers. These steps all work to bring the yield for a flat cover to 34%. For a domed watch cover, the improvement is from 4% to 5%, which is a 25% improvement.
Let’s see what the numbers show. In the worst-case scenario, HPA emits 12.3 grams of CO2 per gram of HPA. Manufacturing our flat watch cover emits:
12.3 x 4.6g /0.28 yield = 202g
And the domed watch cover emits:
12.3 x 4.6g /0.04 yield = 1.4kg
These numbers show why crystal sapphire has been called the “black sheep of watchmaking”.7
The use of current low-emission HPA and digital quality control reduces these values to 20g and 138g, respectively. This is even before zero-emission HPA eliminates the cost completely, if not the wastage. The table above shows that the CO2 emissions can be reduced by 22%.
Conclusion
We have seen that the environmental cost of a watch cover can be very high if one does not think about it. Through attention to detail at all process steps, it can be reduced to that from melting the HPA and running the machines if their power sources are not green. This can be applied to other markets and use cases for sapphire, such as LEDs and other applications. We will discuss these cases in a future article.
References
- https://momentumwatch.com/blogs/momentum-blog/whats-the-benefit-of-sapphire-crystal-sapphire-vs-mineral-crystal
- https://www.innovationnewsnetwork.com/high-purity-alumina-sustainably-produced/29593/
- “Scientific_Visual_Eco-audit-watch-sapphire_article” at https://scientificvisual.ch/
- https://www.imarcgroup.com/watch-market
- https://www.sueddeutsche.de/wirtschaft/rolex-luxusuhren-1.5777888
Please note, this article will also appear in the fourteenth edition of our quarterly publication.