Dr Deepak Choudhury, from the Biomanufacturing Technology Group at BTI A*STAR, explains how its newly-developed strategies can scale-up the extraction of extracellular matrices.
The Tissue Manufacturing team under the Biomanufacturing Technology Group (BMT) focuses on developing platform technologies: (a) to extract extracellular matrix (ECM) from cells and tissues; followed by (b) turning extracted ECM into useful formulations (e.g. hydrogels/bioinks/inks) amenable for subsequent processing, and (c) developing scale-up printing processes/printing platforms, hence manufacturing tissue products. One core platform includes a decellularisation reactor for extracting biomaterials from animal, plant and fungi samples. Our printing know-how includes Roll-to-panel (R2P) and Roll-to-roll (R2R), as well as stereolithography and bioprinting tools. Currently, at BMT, hydrogels, dermal tissue templates, and cultured meat prototypes are being manufactured.
Biomanufacturing dECM
The ECM comprises a complex cocktail of growth factors, hormones, and other proteins that provide biophysical, mechanical, and biochemical signals to the cells.1 This ECM consists of fibrillar, structural, and signalling proteins such as collagen, laminin and fibronectin, glycosaminoglycans (GAGs), proteoglycans, and matricellular proteins.1 The ECM is organ-specific, and its detailed composition varies across organs.1 Being highly bioactive, the ECM can be used to induce cellular activity such as growth, differentiation, migration, and cell organisation in tissues.
Organ-derived decellularised ECM (dECM) bioinks have recently emerged as a good candidate for many biomimetic bioinks.1 dECM can be exploited in bioinks to provide cells with natural microenvironmental niches and complex cues to direct cellular processes, which regular hydrogels cannot. The method to obtain dECM, known as decellularisation, is a recent laboratory process with the associated technologies and devices being largely non-specific, undefined, and meant for lab-scales. There are hardly any commercially available devices explicitly developed for decellularisation.2
A large amount of research has been conducted using whole animal organs’ perfusion, with the goal of recellularisation to obtain functioning organs that potentially reduce the need for allogeneic transplants.2 These involve controlling the flow of detergents and reagents perfusing through the organs and engineered unique protocols to provide oscillating pressure or osmotic shock to decellularise organs. The alternative to whole organ decellularisation is a less explored method of using tissue samples or smaller denominations from animal organs (like minced samples). Most of these methods follow a similar agitation process.3
However, for manufacturing considerations of decellularised tissues, controls need to be in place to ensure consistency and reproducibility. These controls would ensure similar quality and quantity of decellularised product between batches.
We have developed a semi-automated system, namely a decellularisation reactor (dECM reactor, see Fig. 1A), which is geared towards the scalable decellularisation of animal tissues. The dECM reactor adapts a standard decellularisation method (agitation) and uses uniform tissue denominations from animal organs, which are obtained from various animal organs such as heart or liver, and which are cut into cubes or slices. This dECM reactor and the associated process is designed in such a way to allow the progress of decellularisation to be monitored. The loading of tissue samples, reagent, decellularisation, and monitoring of progress all occurs within one reactor, which minimises the number of and movement between types of equipment.
We have decellularised a few hundred grams of porcine tissue samples in one cycle run of the dECM reactor. This decellularised tissue could be freeze-dried to obtain dECM powder (see Fig. 1B) which is subjected to acid hydrolysis to produce a hydrogel. However, as dECM bioinks are inherently soft hydrogels, they would need the support of synthetic materials to make load-bearing scaffolds. Alternatively, chemical modifications and other crosslinking agents, can be used to obtain the desired mechanical properties. We have also adopted a specific chemical modification to crosslink the dECM hydrogel (see Fig. 1C), also ensuring tunable mechanical stiffness and biological functionality. Currently, the dECM reactor is being adapted for decellularising other animal and human tissue samples.
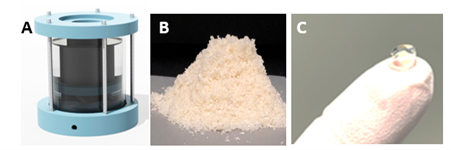
Biomanufacturing cell-derived matrices (CDMs)
Cell-derived matrixes (CDMs) are made of cultured extracellular matrixes (ECMs) which play an essential role in native ECM, influencing cell growth and function, providing an alternative to dECM.4 CDM derived from cell-cultured ECM consist of various growth factors, matrix proteins and macromolecules that make it an excellent replacement for in vivo ECM microenvironment. It can be xeno-free and serum-free, making it a superior choice of a matrix for human cell culture than commonly used animal-derived matrices that may have biocompatibility, ethical, and sustainability concerns.
CDM is easy to acquire by simple monolayer cell culture, involving many flasks and dishes. One can choose the cell type, the culture system for decellularisation (2D vs. 3D), and the cells can be genetically manipulated to increase or decrease specific components. Mesenchymal stem cells (MSCs) are a common source of CDM due to their capability to secrete, deposit, and organise secreted ECM.
CDM and dECM bear similarities in that the ECM collected would have similarity to the source tissue or cells it is derived from. However, a significant difference would be the structural and mechanical characteristic of the ECM obtained. A full organ’s dECM scaffold can be obtained through careful and thorough perfusion decellularisation. CDM, however, does not possess any structural or mechanical traits apart from its chemical composition. However, a benefit of CDM over dECM is its customisability. CDM allows for a high level of customisation of the ECM generated as there is a greater control over the entire process. Cell sources and culture methods can be engineered to tailor the secreted ECM for specific actions. In applications where ECM macrostructure is unnecessary, CDM presents a potentially viable source of ECM.
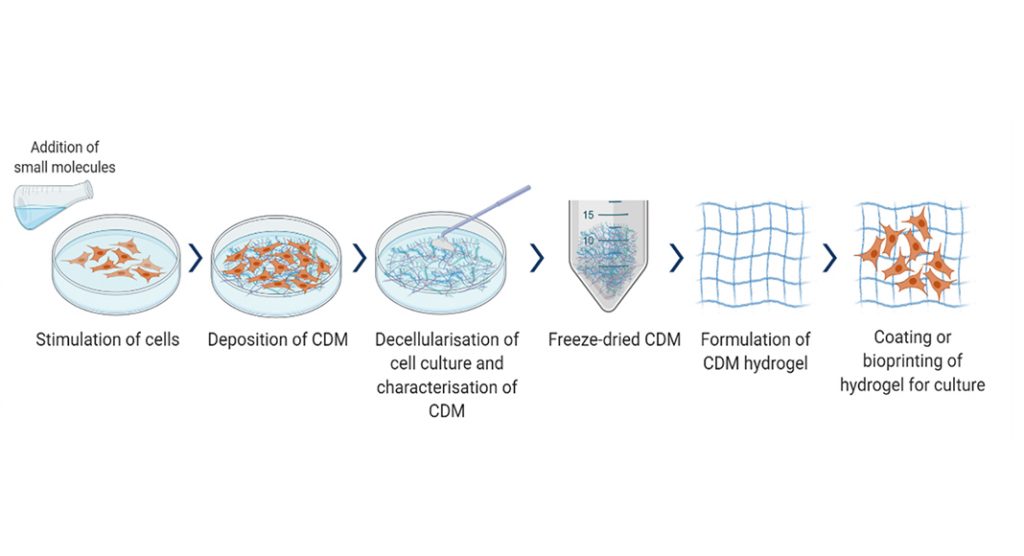
CDMs could be used for various applications:
- As a substrate surface for new cells;
- To form cell sheets that can be arranged to create complex tissue constructs; and
- As a CDM powder to make hydrogels and potentially bioinks.
CDM development is crucial for research and development involving continuous cell cultures, such as regenerative medicine and cell therapy. Current CDM methods from monolayer cell culture are unsustainable due to laborious processes, low yield, and throughput in a few micrograms. At BMT, we have identified strategies that can increase CDM yield through small molecule interventions, optimise extraction and recovery, and develop a scale-up platform to produce a cost-effective supply of CDM. The extraction process parameters are being optimised, keeping in line with a stable and scalable manufacturing process. Our CDM hydrogel will be based on unique cell types and formulations.
References
- Choudhury, H. W. Tun, T. Wang, M. W. Naing,. ‘Organ-Derived Decellularized Extracellular Matrix: A Game Changer for Bioink Manufacturing?’ Trends in Biotechnology 36(8) (2018) 787-805
- Choudhury, M. Yee, Z. L. J. Sheng, A. Amirul, M. W. Naing. ‘Decellularization systems and devices: State-of-the-art’, Acta Biomaterialia 115 (2020) 51-59
- Skardal, M. Devarasetty, H. W. Kang, I. Mead, C. Bishop, T. Shupe, S. J. Lee, J. Jackson, J. Yoo, S. Soker, A. Atala. ‘A hydrogel bioink toolkit for mimicking native tissue biochemical and mechanical properties in bioprinted tissue constructs’, Acta Biomater 25 (2015) 24-34
- T Hoshiba. ‘Cultured cell-derived decellularized matrices: a review towards the next decade’, Journal of Materials Chemistry B 5(23) (2017) 4322-4331
Please note, this article will also appear in the fifth edition of our quarterly publication.