Professor Pierre Sokolsky of the Telescope Array Project speaks to The Innovation Platform about the significance of laboratory expansion in finding the source of high energy cosmic rays.
Extraordinarily energetic subatomic particles are bombarding our atmosphere. Where they come from is a mystery. A newly expanded observatory may give us the answers, explains Pierre Sokolsky, for the Telescope Array Collaboration.
The Earth is constantly bombarded by a stream of cosmic rays – subatomic particles with an enormous span of energies. At the low end, they come from violent events in our galaxy as well as on the Sun’s surface. At the high end, each subatomic particle has the energy of a fast pitch baseball or world-class tennis serve. We know very little about where they come from or what natural processes can lead to such extraordinary energies many orders of magnitude higher than what can be produced at the CERN collider or any feasible future accelerator. Their nature and origin represent one the major mysteries of astrophysics.
Energetic cosmic rays at such extreme energies are rare, on the order of a few particles per square kilometre of Earth per century, necessitating detectors with enormous areas in the thousands of square kilometres. But both because they are so low in rate and because they are blocked by the Earth’s atmosphere, it is impractical to detect them directly on a satellite or a balloon-borne instrument. Indeed. due to their rarity, their detection has to be indirect.
Ultra-high energy cosmic ray particles, mainly protons and lighter nuclei, bombard the upper atmosphere of the Earth and interact with nitrogen and oxygen nuclei to produce cascades of secondary particles in a manner similar to a nuclear chain reaction. The number of secondary particles grows as the cascade progresses through the atmosphere until the particles hit the ground. In the process, excited nitrogen molecules in the atmosphere produce ultra-violet fluorescence light, which can be detected by specialised telescopes, first developed at the University of Utah for the Fly’s Eye cosmic ray detector in the 1980s.
Simultaneously, nuclear detectors spread out on the ground can detect the last shower particles. The combination of air-fluorescence telescopes and surface detectors provides a powerful hybrid method for extracting the maximum amount of information from the interaction of these enigmatic visitors from beyond our galaxy with our atmosphere, including particle energy and arrival direction.
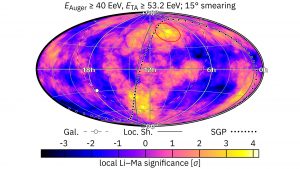
The extreme nature of the acceleration
A major reason for scientists’ interest in this radiation is the extreme nature of the acceleration process that must produce it. There are few viable mechanisms. These include:
- Matter ejected in the jets produced by super-massive black holes in the centres of galaxies;
- Processes in so-called ‘starburst galaxies’ where new stars are being created at a high rate; and
- The collapse of some types of very massive stars leading to extraordinary releases of energy dubbed gamma ray bursts (GRBs).
Simplification
It may appear that in our huge Universe, with numberless galaxies of various sorts extending to the edge of what we can detect, identifying particular sources just from pointing back to the celestial sphere would lead to too many possibilities for useful identification. However, a significant simplification occurs due to the existence of the primordial black-body radiation left over from the Big Bang that permeates all space. It turns out that at the highest energies, cosmic ray particles will interact with the photons in this radiation and lose energy.
A major prediction of the exact nature of this interaction was made in the 1960s: the number of protons above the so-called GZK energy of 3×1019 eV will be suppressed. In other words, the cosmic ray flux will not extend forever in energy but be cut off. This was in fact first observed by the High Resolution Fly’s Eye experiment, a predecessor to the currently active observatories. This means that the highest energy particles could not have travelled too far through space. In fact, their sources must lie somewhere in the local super-cluster of galaxies centred on Virgo within about 100 million parsecs (300 million light years) from Earth. In a sky map, these galaxies look like an extended region along what is called the super-galactic plane (see the dotted line in Fig. 1). A major question is: do the highest energy cosmic rays really point back to this plane and to particular galaxies known to produce lower energy photons, neutrinos, or even gravitational waves?
A confounding difficulty is that cosmic rays are electrically charged and therefore bend in cosmic magnetic fields. At lower energies, the presence of these fields in our galaxy and in extra-galactic space means that arrival directions are scrambled relative to sources due to particle bending. However, at the highest energies, this effect is minimised, particularly if the particles are protons or light nuclei, and sources should begin to stand out.
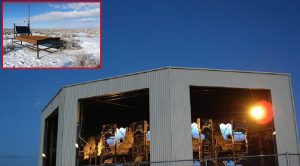
The Telescope Array (TA) project
In recent years several international observatories have begun to make progress is solving this mystery. The Telescope Array (TA) project, a consortium of US, Japanese, Korean, Russian, and European scientists, have deployed sophisticated detectors over thousands of square kilometres of desert in the US state of Utah to capture these rare events and determine their energy, composition, and source direction in the northern sky. Another international collaboration operates a similar detector called the Pierre Auger Observatory (PAO), in Mendoza province in the pampas of Argentina, observing the southern sky.
Clues have begun to emerge from the data taken by these observatories – the highest energy particles are arriving from sources far beyond our galaxy, clustered near Ursa Major in the north and the galaxy Centaurus A in the south, with indication of other sources along the supergalactic plane containing many of our nearby galaxies (see Fig. 1).
Enhancing sensitivity
Encouraged by these emerging clues, both observatories have embarked on ambitious enhancements and expansion of their detectors to obtain better sensitivity to the sources. TA is in the throes of an expansion to what is known as TAx4 – a 3,000 square kilometre array of surface detectors overlooked by five stations of air fluorescence telescopes (see Fig. 2). The PAO observatory, also at 3,000 square kilometres, is enhancing the surface detectors to better determine the composition of these particles (Auger Prime). Are they protons, nuclei such as carbon, nitrogen or even Iron? Do these particles interact in new ways, unseen with the lower energy experiments conducted at CERN and other Earth-bound accelerators? Since protons are bent less than heavier nuclei by magnetic fields, will selecting protons from the mix allow us to see the location of the sources more clearly?
Combining data from neutrino telescopes such as IceCube in Antarctica, the new gamma-ray detectors such as CTA (now being constructed), and gravitational wave detectors such as LIGO and VIRGO also opens up a new way of examining the nature of cosmic rays. Cosmic ray sources may simultaneously produce particles and waves that these detectors are sensitive to.
This ‘multi-messenger’ approach that is now becoming possible is the result of a new generation of ultra-high energy cosmic ray and other detectors poised to finally illuminate the nature and origins of this extraordinary radiation from some of the most violent processes in the Universe.
Professor Pierre Sokolsky
Telescope Array Project
Department of Physics and Astronomy
University of Utah
+1 801 209 1011
ps@physics.utah.edu
www.telescopearray.org
Please note, this article will also appear in the second edition of our new quarterly publication.