Professor Marek Gazdzicki discusses SHINE – a high energy nuclear and elementary-particle physics experiment located at CERN that explores heavy-ion collisions, neutrinos, and cosmic rays.
SHINE is a special place where the physics of heavy-ion collisions, neutrinos, and cosmic ray meet. This high energy nuclear and elementary-particle physics experiment is located at the Super Proton Synchrotron of the European Organization for Nuclear Research (CERN) in Europe. Here, for more than 10 years, the three physics communities have gathered to conduct numerous measurements urgently needed to answer important questions:
- What happens to matter created in heavy-ion collisions when its volume and density change?
- What happens in neutrino production targets of long-baseline neutrino oscillation experiments?
- What happens to cosmic rays passing through the Earth’s atmosphere and intergalactic medium?
The unprecedented variety of beam particles – from the lightest pions to heavy nuclei of lead atoms – together with a possibility to vary their energy in a broad range makes the Super Proton Synchrotron with its North Area beamlines a world-unique facility. The SHINE detector has greatly profited from a long tradition in and synergies of particle physics experiments at CERN. It allows for precise measurements of hadrons produced in collisions of beam particles with various nuclear targets. Needless to say, these experimental opportunities attract physicists from all over the world. By bringing together their knowhow and resources, they can reach different physics goals with very high efficiency. This would not be possible without the close collaboration between different physics communities within SHINE.
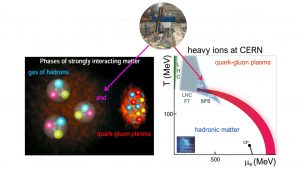
The first period of SHINE measurements started in 2009 and was completed in 2018. Now, during the CERN’s second Long Shutdown, a major detector upgrade is being conducted. The second period of physics measurements is set to begin in October this year and it will continue until the beginning of Long Shutdown 3 at the end of 2024. Plans concerning measurements during the period 2026-2029 are under discussion.
The physics of strongly interacting matter
One of the important issues in contemporary physics is the understanding of strong interactions and, in particular, the study of the properties of strongly interacting matter – a system of strongly interacting particles in equilibrium. The advent of the quark model of hadrons and the development of the commonly-accepted theory of strong interactions – quantum chromodynamics (QCD) naturally leads to expectations that with increasing energy density the matter undergoes a transition from a gas of hadrons to a state of quasi-free quarks and gluons – the quark-gluon plasma. The nature of these phase transitions is studied by SHINE by colliding atomic nuclei.
SHINE measurements for the physics of strongly interacting matter started with the unique two-dimensional scan in collision-energy and mass of nuclei. The scan was needed to find out how the signals of the beginning of a quark-gluon plasma creation observed at the intermediate SPS energies in lead-lead collisions evolve when masses of colliding nuclei decrease. The results are surprising: one observes a rapid change of light-hadron production properties between collisions induced by beryllium and argon beams. The effect is referred to as the onset of a fireball – the beginning of the formation of large-volume strongly interacting matter. Detailed measurements in the onset of the fireball region are needed and are planned to be conducted after the Long Shutdown 3.
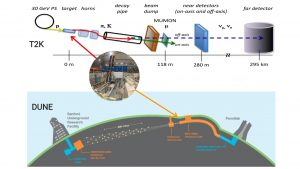
In the coming years, SHINE will perform pioneering measurements of charm hadrons in lead-lead collisions. They will be possible thanks to the major detector upgrade leading to a ten-fold increase in the data acquisition rate and a very high precision (below 200 micrometers) of finding decays of short-lived charm hadrons.
The data recorded with the two-dimensional scans are used to search for the deconfinement critical point of strongly interacting matter – a hypothetical end point of the first-order transition line to the quark-gluon plasma that has properties of a second-order phase transition. A possible observation of a critical point signal may strongly impact future plans of SHINE.
Measurements for neutrino physics
The main goal of long-baseline neutrino oscillation experiments is to study transitions between neutrinos of different types: electron, muon and tau. Nowadays, key questions driving the field concern the neutrino mass hierarchy and the CP violation in neutrino oscillations.
Neutrino beams are produced in a two-step process. Firstly, a very high intensity proton beam impacts a solid target about a metre long, and many hadrons (mostly pions, kaons and protons) are produced. Secondly, charged pions and kaons are focused by magnetic fields and decay. Among decay products are neutrinos, which fly through the Earth’s crust to huge detectors placed many hundreds of kilometres away from laboratories where neutrino beams are produced. There, a flux of neutrinos of different types is measured.
By comparing this flux to the initial flux, one extracts information on the neutrino properties. However, it is not easy to measure the initial neutrino flux with sufficient precision. To overcome this problem, the targets for long-baseline neutrino experiments can be placed in the SHINE detector, which can make precise measurements of the types and energies of the particles that emerge from the target – information that can be used to predict the neutrino beam’s characteristics. SHINE has to adjust the proton beam of properties to the ones of the corresponding neutrino experiment.
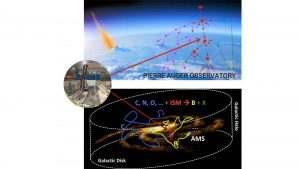
This type of SHINE measurement was initiated in 2007 in collaboration with the T2K experiment at J-PARC in Japan. The SHINE data greatly improved the precision of the final T2K results. The second phase of the measurements started in 2015 in collaboration with the long-baseline experiments at Fermilab in the USA. The measurements for the future neutrino oscillation experiments T2HK at J-PARC and DUNE at Fermilab will be performed within the next several years and might continue even after CERN’s Long Shutdown 3. Moreover, an upgrade to the SHINE beamline by constructing a very low-energy branch is being discussed. This would allow to provide missing data to several other neutrino experiments.
Measurements for cosmic-ray physics
Many important physics questions can be addressed by studying high-energy protons and atomic nuclei which fly across cosmic space. They are referred to as cosmic rays and their properties are measured using various strategies.
First, when impacting the Earth’s atmosphere they produce showers of particles which can be detected by particle detectors placed on the ground. The data allows the reconstruction of properties of the cosmic-ray particle which initiated the shower. This requires detailed simulation of particle interactions which dominate the shower development. This strategy has been followed by the Pierre Auger Observatory in Argentina and KASCADE in Germany. Requests of these experiments motivated SHINE measurements for cosmic-ray physics. Precise information on interactions of pions – the most numerous hadrons in air showers – has been provided by SHINE.
Second, cosmic rays can be measured directly by installing particle detectors on satellites, like the AMS detector on the International Space Station. Recent data from these experiments provide a unique diagnostic of cosmic-ray propagation in the Galaxy and an opportunity to find signatures of astrophysical dark matter annihilation. However, the current uncertainties in the modeling of the propagation of cosmic rays in the Galaxy are dominated by uncertainties of data on interactions of cosmic-ray protons and nuclei with interstellar medium protons. To remedy this situation, SHINE is performing new precise measurements of interactions of light nuclei on protons.
The SHINE programme beyond CERN’s Long Shutdown 3 is now under discussion. The versatility of the CERN facility and the unique properties of the SHINE detector motivate and inspire many ideas. They will be presented and considered at a dedicated workshop due to take place this year. The outcome of this process will lead to the proposal of new measurements as well as necessary detector and facility upgrades.
Acknowledgements
This work was supported by the Polish National Science Center 2018/30
/A/ST2/00226 and the German Research Foundation GA1480/8-1.
Please note, this article will also appear in the sixth edition of our quarterly publication.