The passive design philosophy used in small modular reactors can create a safer, more economical future for nuclear power – but proof is essential!
Energy production lies at the foundation of modern society. By powering industries and technologies that have dramatically increased worker productivity, much of the world has achieved a higher quality of life in the present day than at any other time in human history. The cost, however, will be steep: The vast majority of energy has been produced by burning various fossil fuels such as coal, oil, and natural gas, releasing immense quantities of carbon dioxide into the atmosphere, as well as a plethora of carcinogenic and asthma-inducing substances. The direct health impact of the latter notwithstanding is that carbon dioxide acts as a greenhouse gas, trapping the energy of solar radiation and warming the atmosphere.
This process, referred to as global warming, represents an existential threat to the majority of life on our planet – ourselves and our way of life included. That carbon dioxide acts as a greenhouse gas, that the release of carbon dioxide by human industrial activity has induced global warming, and the general catastrophic implications of global warming for life on Earth are a matter of virtually unanimous consent by the scientific community.¹
Small modular reactors: An innovative solution?
In an effort to halt additional damage to the environment and with an eye to improving energy security, many nations are rapidly researching and adopting carbon-neutral energy policies. This has led to a renewed interest in nuclear technologies, manifesting in research spanning the entire spectrum of the field, from advanced Generation-IV reactor designs to accident-tolerant fuels and closed-fuel cycles.
A common thread of this resurgence, however, is a trend towards smaller designs, which, it is hoped, will overcome the difficulties faced by the nuclear industry in commissioning new plants. Project C1 within ANItA, a Swedish national competency centre for nuclear power technology, seeks to expand knowledge surrounding the safety of such smaller designs, termed small modular reactors (SMRs).
SMRs, defined broadly by the IAEA as reactors with power capacities up to 300 MWe that can be factory fabricated and transported as modules², offer several distinct advantages over their larger counterparts.
Firstly, large reactors are not a realistic option for many localities; by reducing the size and cost, SMRs provide flexibility, allowing for the introduction of nuclear power to regions that do not need the immense output of large reactors. Next, SMRs generally enjoy much simplified designs thanks to their smaller, more easily cooled cores; this, combined with their factory fabrication, can reduce construction times.
Finally, these factors combined can help reduce their cost by minimising interest payments and time without revenue, mitigating the reduced economy of scales inherent to using a smaller design. Whether these factors will make SMRs competitive with large reactors will likely depend on the region of interest and the ability of the manufacturers to scale production.³
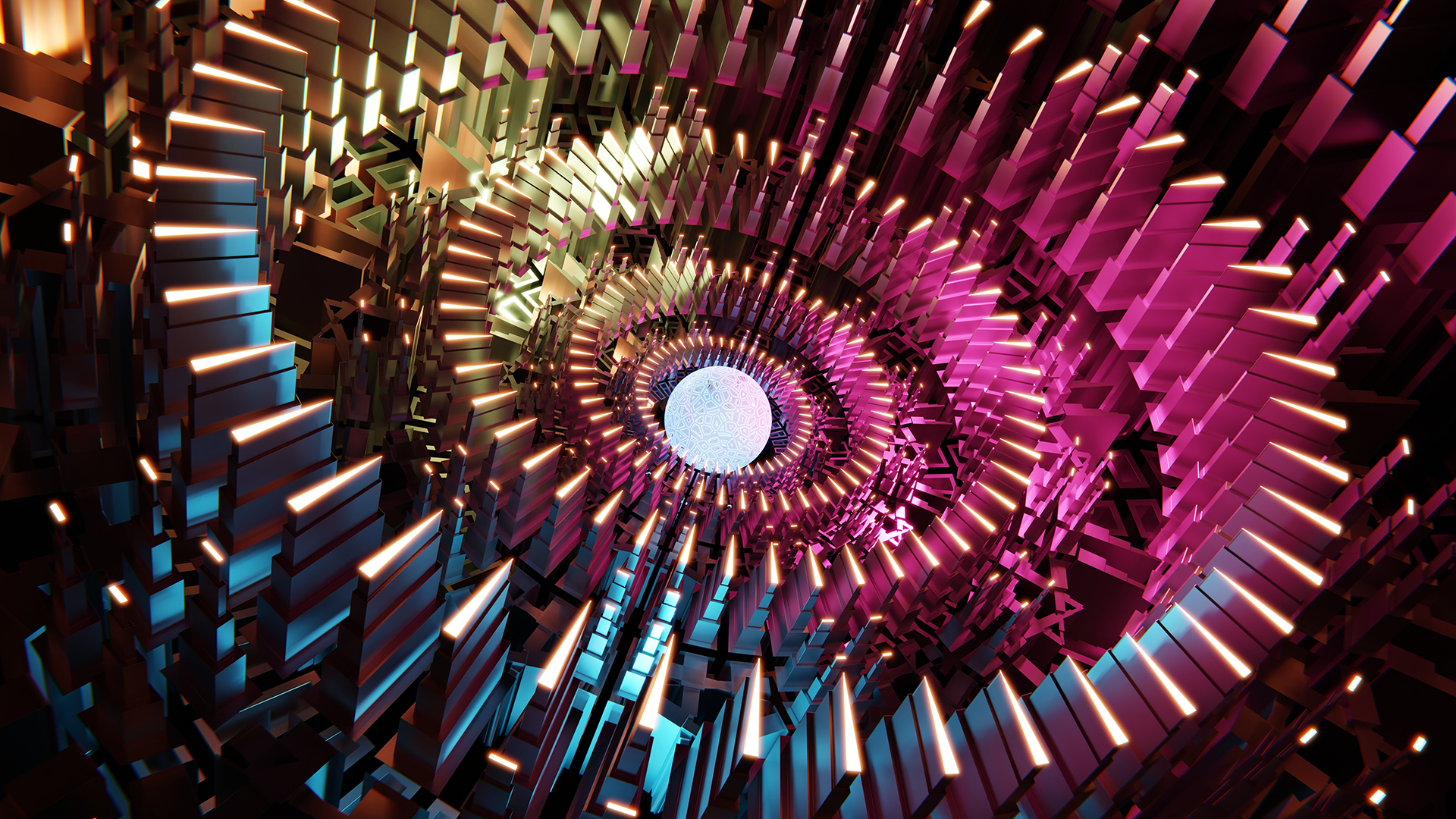
The focus of ANItA Project C1, however, centres on the fact that their smaller energy output allows for the shutdown core to be cooled entirely using passive systems which rely on natural circulation. This is in contrast to most Generation III and older plants, which rely on active systems (e.g., pumps) to remove decay heat from the core after the reactor is shut down.
While some large, Generation III+ designs adopt the use of passive cooling systems (see the AP1000 and ESBWR), the inclusion of passive systems is practically universal among light water SMR concepts.²
It is typically believed that this design philosophy will further enhance safety,⁴ ensuring that the risk of a severe accident is reduced and justifying changes in plant siting that could enhance the economics of SMRs. This includes co-siting many reactors adjacent to one another or locating plants closer to population centres for the purpose of district heating and producing clean water through desalination.
However, this will require new regulations and licensing practices adopted for SMRs, necessitating rigorous scientific evidence backing up the claims of enhanced safety. Evaluating such evidence is one goal of ANItA, with research in this project being conducted at the Royal Institute of Technology in Stockholm, in collaboration with Vattenfall, Westinghouse, and the rest of the ANItA competency centre. On this basis, a discussion about passive systems and natural circulation is warranted.
Passively safe through natural circulation
A passive system is defined by the IAEA as either a system which is composed entirely of passive components and structures, or a system which uses active components in a very limited way to initiate subsequent passive operation.⁴ While conceptual SMR designs utilise a number of different passive features to improve safety, such as accident-tolerant fuels, virtually all light water SMR designs utilise natural circulation in some fashion as a passive mechanism to accomplish their safety objectives.
Because passive systems do not rely on an active power source, they are generally considered more reliable than their active counterparts. However, the methods with which the reliability of a system is most often evaluated, e.g., using fault tree analysis with historical data for failure rates of active system components, can be difficult to apply to passive systems.
First, there is no such set of historical data to turn to for assessing the failure rate of some passive functions. Second, what constitutes passive system failure may not be as well defined, as a passive mechanism may operate between what is traditionally defined as success or failure. Third and finally, the applicability of current reactor system codes to model passive systems for the purpose of safety analysis and licensing is still an open question.⁵
These points readily apply when considering the natural circulation-driven cooling systems used for the core and containment of many SMR designs, which operate on the principle of buoyancy-driven flow. Plainly speaking, hotter water and steam are less dense and so will tend to flow up, whereas colder water is more dense and will tend to flow down; therefore, by placing the heat sink (e.g., a heat exchanger which will transfer heat to the atmosphere) above the heat source (e.g., the reactor core, or another heat exchanger if the system is to cool the containment), we can ensure flow from the latter to the former, accomplishing the function of a pump without an external source of power.⁶
Thus, while there are component failure rates for parts of the system crucial for establishing system flow, such as valves that must be opened or heat exchangers which cannot be obstructed, natural circulation itself does not have a traditional ‘failure rate.’
Additionally, even if sufficient flow is not established during a casualty to prevent damage to the plant, some limited flow may still exist, which can slow down accident progression, give additional time for accident management, and limit damage to the plant. Finally, while system codes are capable of simulating natural circulation, the results are generally less accurate compared to models for forced circulation systems.
While these concerns are themselves legitimate, one may nonetheless ask, ‘Why does this matter? If flow by natural circulation is guaranteed, why bother assigning a failure rate, and what difference do slight modelling errors make?’ A possible concern regarding natural circulation is its susceptibility to flow instabilities, especially at lower system pressures; essentially, the flow rate can oscillate, or even reverse, in a chaotic fashion.⁷
Further, these oscillations may occur as a result of variations in a large number of system parameters, many of which will be different depending on when the associated system is needed. Assessing the impact these oscillations can have on the safety function of a natural circulation system will be necessary for their widespread adoption.
Ongoing research
Resolving these overlapping challenges will require a multifaceted approach. To briefly summarise the research goals of ANItA project C1:
Validate system codes
By simulating thermal-hydraulic experiments and tests conducted on operational nuclear power plants with deterministic codes used in safety analysis, we can improve the models and assess uncertainties of predicted natural circulation flow rates and heat removal efficiency in order to realise sufficient safety margins in SMR designs that rely on natural circulation.
Assess the reliability of natural circulation systems With a level of confidence established in our codes, we can model safety systems driven by natural circulation and evaluate their reliability. This can be achieved through various methods, such as conducting multiple simulations while varying system parameters that carry some uncertainty – like confidence intervals related to material property measurements. Using statistical methods and probabilistic safety codes, we can estimate the probability of system failure while also testing the design’s robustness.
Analyse a generalised boiling water SMR
In addition to only modelling a passive safety system, it would be enlightening to evaluate an entire SMR design to compare their safety to their larger counterparts. In this case, a boiling water reactor would be most interesting, as newer designs – such as the ESBWR and BWRX-300, both by GE Hitachi – utilises natural circulation in lieu of recirculation pumps to drive flow through the core during normal operation. Testing the stability and load-following characteristics of such systems, as well as performing a full safety analysis, would provide both academic and industrial value.
Compiling the results of this research will provide designers, regulators, policymakers, and researchers in adjacent fields with the necessary context to form an educated opinion on the reliability of natural circulation-based passive safety systems. It is hoped that this work will form a foundation for improving light water reactor safety and facilitate an efficient and timely implementation of new nuclear power within Sweden.
References
- “Climate Change 2021: The Physical Science Basis,” 2021, Intergovernmental Panel on Climate Change. [Online]. Available:
https://report.ipcc.ch/ar6/wg1/IPCC_AR6_WGI_FullReport.pdf - “Advances in Small Modular Technology Developments: 2022 Edition,” 2022, International Atomic Energy Association. [Online]. Available: https://aris.iaea.org/Publications/SMR_booklet_2022.pdf
- B Mignacca and G Locatelli, “Economics and finance of Small Modular Reactors: A systematic review and research agenda,” Renewable and Sustainable Energy Reviews, vol. 118, p. 109519, Feb. 2020,
doi: 10.1016/j.rser.2019.109519. - “Passive Safety Systems and Natural Circulation in Water Cooled Nuclear Power Plants,” 2009, International Atomic Energy Association. [Online]. Available: https://www-pub.iaea.org/MTCD/Publications/PDF/te_1624_web.pdf
- L Burgazzi, “Addressing the challenges posed by advanced reactor passive safety system performance assessment,” Nuclear Engineering and Design, vol. 241, pp. 1834–1841, 2011, doi: 10.1016/j.nucengdes.2011.02.002.
- T Houghton and D Wilson, “Natural Convection and Passive Cooling,” in Nuclear Heat Transfer and Passive Cooling, vol. 3, Frazer-Nash Consultancy, 2021. Accessed: Nov. 13, 2023. [Online]. Available: https://www.imeche.org/docs/default-source/1-oscar/industries-and-specialisms/nuclear/vol3_passivecooling_issue1_dec2021.pdf?sfvrsn=2
- U Rohatgi and R Duffey, “Natural circulation and stability limits in advanced plants: The Galilean law,” presented at the International conference on nuclear systems thermal hydraulics, United States, Jun. 1994. [Online]. Available: https://www.osti.gov/biblio/10137311
Please note, this article will also appear in the 20th edition of our quarterly publication.