Jon Lapington, Professor of Space Physics and Head of the High-Speed Imaging Group at the University of Leicester, and Paula Chadwick, Professor of Astrophysics and Head of the Physics Department at Durham University, discuss the UK’s role in very-high-energy gamma-ray science and the prospects for future leadership.
Astroparticle physics is a rapidly expanding field lying at the crossover between particle physics, astrophysics, and cosmology. It is the study of fundamental particles and fields of astrophysical origin and, over recent years, has driven multi-messenger astronomy – a powerful extension to conventional astronomy, where information from two or more of the four cosmic ‘messengers’ (electromagnetic radiation, gravitational radiation, neutrinos, and cosmic rays) are combined to provide insights into the physics of extreme events and the ultimate nature of matter. These messengers, produced by events such as supernova explosions and outbursts around black holes, provide complementary information about cosmological sources and populations.
Major achievements in recent years include the discovery of gravitational waves produced by black hole and neutron star mergers;¹ the discovery of the diffuse background of very-high-energy neutrinos from the Milky Way;² and the first detection of PeVatrons, sources of photons exceeding 1 PeV in energy,³ representing a new era in ultra-high-energy gamma-ray astronomy.
A great advantage of using multi-messenger observations is the varied nature of the science that can be performed – from the physics of jets and particle acceleration to fundamental questions of the nature of gravity and dark matter. These scientific returns are best accomplished by a combination of measurements rather than by any single experiment or observatory.
The UK community has long and substantial involvement across the breadth of astroparticle physics – including very-high-energy (VHE, E > 10GeV) gamma-rays, ultra-high-energy cosmic rays, gravitational waves, neutrino astrophysics and direct dark matter searches – which places us in an enviable position to make best use of multi-messenger astronomy to produce science that is greater than the sum of its parts.
VHE gamma-ray science
VHE gamma-ray astronomy, one of the astroparticle physics themes, began with the creation of the first atmospheric Cherenkov telescope by John Jelley and Bill Galbraith in the unlikely surroundings of AERE at Harwell in Oxfordshire.⁴
From those early beginnings, it has become a field of active research in the UK with several groups collaborating in the development of ground-based VHE gamma-ray observatories capable of detecting extremely high-energy events beyond energies achievable in terrestrial particle colliders.
In the current decade, the number of dedicated observatories providing VHE gamma-ray data is set to grow substantially. The Chinese Large High Altitude Air Shower Observatory (LHAASO)⁵ began observations in 2020, and the decade will see the commissioning of two further next-generation astronomical facilities: the Cherenkov Telescope Array Observatory (CTAO), an Imaging Atmospheric Cherenkov Telescope (IACT) array slated to come on stream from 2025 onwards,⁶ and the Southern Wide-field Gamma-ray Observatory (SWGO),⁷ a Water Cherenkov Detector (WCD) array, expected a few years later.
All these observatories rely on detecting the faint, rapid flashes of blue Cherenkov radiation created by the charged particles produced in the upper atmosphere by incoming gamma-rays: for LHAASO and SWGO, the Cherenkov radiation is produced by capturing the particles in purified water, while CTAO detects the Cherenkov light produced by the particles in the atmosphere. The latter two facilities, having sites in the southern hemisphere, have the great advantage of viewing the centre of the Galaxy – a region rich in astronomical objects. UK groups are making significant contributions to both observatories.
The Cherenkov Telescope Array Observatory
The Cherenkov Telescope Array Observatory (CTAO) is currently at the beginning of its construction phase. Comprising two arrays of IACTs, one in the northern and one in the southern hemisphere, it will transform our understanding of the high-energy Universe and explore physics questions of fundamental importance.
Three telescope sizes are required to cover the gamma-ray energy range of the CTAO, which extends from 20 GeV to 300 TeV. The Large-Sized Telescopes are optimised for the lowest energies, their extremely large 23m diameter primary mirror efficiently capturing the lower intensity and smaller footprint Cherenkov light from energies in the range 20 to 150 GeV. For higher energies, the larger array of Medium-Sized Telescopes of 12m diameter is optimised for energies in the range 150 GeV to 5 TeV, while the large array of Small-Sized Telescopes of 4m diameter is optimised for the highest energies, from 5 TeV and above.
CTAO’s more than 60 telescopes will be situated on the island of La Palma in the Canary Islands, and in the Atacama Desert, Chile. This major new, ground-based VHE gamma-ray facility is backed by a global community, being top-ranked in ASTRONET⁸ and fully endorsed by the Astroparticle Physics European Consortium (APPEC).⁹
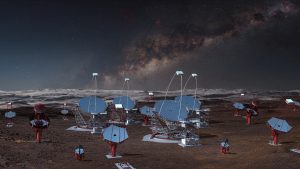
UK contribution to the Small-Sized Telescopes
The array of 37+ Small-Sized Telescopes (SSTs) at the CTAO southern site will provide unprecedented sensitivity above 5 TeV, and will offer the highest angular resolution of any instrument at these energies. Following a down selection from three prototype telescopes, the design finally selected for the SSTs comprises a dual mirror Schwarzschild-Couder10 optic with a 4.3m diameter primary mirror and a 1.8m secondary mirror. The dual mirror optics produce a ~9° field of view with a smaller plate-scale aplanatic focal plane allowing a small (~40cm diameter), low-cost silicon photomultiplier (SiPM)-based camera to be employed, compared to the larger cameras (~2m diameter) based on photomultiplier tubes (PMTs) required for conventional single mirror Davies-Cotton11 IACT designs.
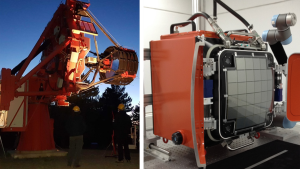
A collaboration comprising the universities of Leicester, Durham, Liverpool and Oxford working with UK industry has been central to the design of the camera for the SSTs. The result is a photon-counting imaging detector with sub-nanosecond time resolution capable of imaging the footprint of typically a few tens of photons of Cherenkov light against a background of starlight which may be hundreds of millions of photons per second in every camera pixel.
The camera comprises an array of 2048 SiPM pixels, configured as 32 sensor and electronics modules each with an 8 × 8 pixel² tile populated with 6 × 6mm² SiPM pixels. Full waveform capture on every camera pixel is provided by the TeV Array Readout Electronics with GSa/s sampling and Event Trigger (TARGET) ASIC which performs the dual function of event triggering and waveform digitisation of the full camera at 1 GSample/s.11
The manufacture of the first cameras for the CTAO construction phase has begun, and the first SST telescope will be commissioned on site in late 2025.
The Southern Wide-field Gamma-ray Observatory
The SWGO comprises an array of Water Cherenkov Detectors (WCD) which are situated at high altitude > 4500m above sea level. Cherenkov photons are produced within the WCD from the direct interaction of particles of the extensive air showers produced by gamma-rays or cosmic-rays with the water. Unlike IACT arrays, which have modest field of view and operate only at night, SWGO has a very large field of view of ~steradian and close to a 100% duty cycle. This makes it particularly suited to mapping of large-scale emission and searching for transient and variable multi-wavelength and multi-messenger phenomena. The IACTs, with their better energy and angular resolution, are more suited to detailed studies of high-energy phenomena, making the CTAO and SWGO highly complementary.
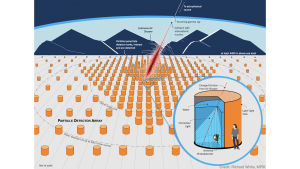
UK involvement in SWGO includes testing and calibration of PMTs including research into the possibility of using wavelength shifting materials with smaller, cheaper PMTs to reduce costs, and the development of calibration light sources.
UK involvement in VHE gamma-ray science
Multi-messenger astronomy is going from strength to strength, as the breadth of science that can be accessed becomes more apparent. In the next ten years, many experiments and observatories – CTAO, SWGO, LISA etc. – will begin operations. Having already played a leading role in the development of the Small-Sized Telescope, UK participation in the CTAO will be a crucial element in the UK’s world-leading capabilities — from radio to VHE gamma-rays on the EM spectrum plus the non-EM messengers — which place it in a uniquely strong position globally. However, strength in depth must be maintained; funding cuts in recent years threaten areas such as VHE gamma-ray astronomy so that UK leadership has been diminished and even modest participation in the CTAO is under threat. It would be a pity if the country which developed the first atmospheric Cherenkov telescope was not able to participate in the ultimate scientific fruition of the technique.
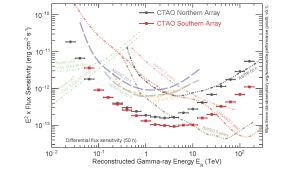
References
- Abbott et al., The Astrophysical Journal Letters, 915, L5 (2021)
- IceCube Collaboration, Science, 380, 1338 (2023)
- Cao, Z. et al. (LHAASO Collaboration), Nature, 594, 33 (2021)
- Galbraith, W, & Jelley, J.V., Nature, 171, 349 (1953)
- Ma, X-H et al. (LHAASO Collaboration), Chinese Physics C, 46, Chapter 1 (2022)
- Science with the Cherenkov Telescope Array, https://arxiv.org/abs/1709.07997 (2017)
- Hinton, J.A. (on behalf of the SWGO collaboration), Proceedings of ICRC, https://ui.adsabs.harvard.edu/abs/2021arXiv211113158H/abstract (2021)
- https://www.astronet-eu.org/?page_id=521
- https://www.appec.org/roadmap/
- Schwarzschild, K., Astronomische Mittheilungen der Koeniglichen Sternwarte zu Goettingen (1905)
- Davies, J. M. & Cotton, E. S., Solar Energy, 1, 16 (1957)
- Lapington, J. et al. (CTA SST Collaboration), Nuclear Inst. and Methods in Physics Research, A,1055, article id. 168433 (2023)
Please note, this article will also appear in the 20th edition of our quarterly publication.