The Muon g-2 experiment at Fermilab is seeking to test the theory of the Standard Model and to potentially discover ‘new physics’. Here, we find out more.
Based at the U.S. Department of Energy’s (DOE) Fermi National Accelerator Laboratory (Fermilab) in Illinois, the Muon g-2 experiment uses Fermilab’s powerful accelerators to explore the interactions of short-lived particles known as muons. The muon is one of the fundamental subatomic particles. Muons offer an insight into the subatomic world and could be interacting with yet undiscovered particles or forces.
A muon is around 200 times as massive as its cousin, the electron. Muons occur naturally when cosmic rays strike Earth’s atmosphere, and particle accelerators at Fermilab can produce them in large numbers. Like electrons, muons act as if they have a tiny internal magnet. In a strong magnetic field, the direction of the muon’s magnet precesses, or wobbles, much like the axis of a spinning top. The strength of the internal magnet determines the rate that the muon precesses in an external magnetic field and is described by a number that physicists call the ‘g-factor’. This number can be calculated with ultra-high precision.
The main goal of the experiment is to test the predictions made by the Standard Model of particle physics. Sensitive detectors installed along the ring will measure the orbits and the precessions of positrons with greater accuracy than ever before — to 140 parts per billion. The results, which will only be known upon the completion of several runs, will be compared to theorists’ g-2 prediction. If the experimental result differs from the theoretical prediction, it could be a sign of undiscovered physics beyond the Standard Model.
The Muon g-2 experiment follows an experiment at the DOE’s Brookhaven National Laboratory, which concluded in 2001. This experiment offered hints that the muon’s behaviour disagreed with the Standard Model. In 2021, the first results from the Muon g-2 experiment were released and showed evidence of muons behaving in a way that differs from that predicted by the Standard Model. This measurement strongly agrees with the value found at Brookhaven.
The Muon g-2 has undergone several data-taking periods, known as ‘Runs’, and is currently in Run 6. Scientists are in the final stages of data analysis for Runs 2 and 3 and are preparing to announce the results later this year.
To learn more about the experiment and what we can expect in the near future, Editor Georgie Purcell spoke to Sean Foster, Research Scientist at Boston University, and Elia Bottalico, Postdoctoral Researcher at the University of Liverpool, who are both heavily involved with the experiment.
Can you briefly summarise the Muon g-2 experiment and its key goals? What implications could the results of the Muon g-2 experiment have on the Standard Model?
Sean Foster (SF): The Muon g-2 experiment has a very singular goal. We are trying to measure one quantity: the anomalous magnetic moment of the muon. This is the strength of the magnetic interaction of the muon. When a muon is placed in a magnetic field, it will carry out some observable actions and we are trying to measure how strongly it couples to that magnetic field.
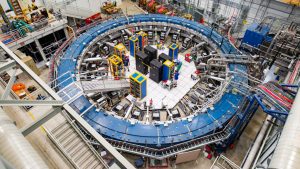
Photo: Reidar Hahn, Fermilab
One of the reasons that the muon is interesting to study is that, when you place it in a magnetic field, one of its properties – known as the ‘spin of the particle’ – undergoes a process called ‘precession’, which means it will start to wobble like a top. In the experiment, we measure the rate of this wobbling, and that observable quantity is determined by the makeup of our Universe. The muon is just one particle of many in our Universe. There are various interactions that exist within our Universe, and all those things come into play to dictate the size of the wobble. Some of these things can have very small effects on this quantity, but they all have an impact. From that perspective, we can measure this quantity and compare it to the theory.
Theorists have devised different theories to describe the Universe – the Standard Model being our best theory yet. Theorists can offer predictions for an anomalous magnetic moment that we can then measure and predict. Doing a comparison of the measurement and prediction can provide a precision test of that theory. If the numbers agree, that will give us confidence in the theory. However, a disparity would suggest that the theory could be missing something. This is exciting as it could lead to the possibility of ‘new physics’.
One thing that I’ve appreciated is the collaborative effort of this experiment. Although we are measuring just one quantity, the Muon g-2, it takes many different inputs from collaborations all over the world to build confidence that this experimental technique is robust. It is intense but it is a lot of fun.
The Muon g-2 experiment has recently celebrated a major milestone after achieving a data highpoint. What is the significance of this?
Elia Bottalico (EB): This is an important goal defined before the experiment started. In particle physics, we begin an experiment with a technical design report (TDR) which outlines a structure of how to develop the experiments.
One of the milestones was in the statistics of the number of events collected. Our goal was 21 BNL – 21 times the number of positrons collected in the previous experiment at Brookhaven National Laboratory (BNL). Carrying out these very precise measurements will offer a lot of information. If we can replicate such experiments with this precision, we can confirm that the way the experiment has been done previously is correct.
As Sean said, we also have to check the theory. In the previous experiment, we found a 3.7 sigma discrepancy with the Standard Model. After Run 1, we found a 4.2 sigma discrepancy. During these years, we also came across another prediction from the theory, which found that the new predicted value is between the Standard Model and the experimental results. Improving our statistical precision will provide a better understanding of what is happening. We now have two puzzles – one between theory and experiment and another one within the theory.
When will the results from Runs 2 and 3 of the experiment be released? What can you tell us about this release?
SF: The Run 2 and 3 data has been under intense analysis for the last few years and are nearing completion. Lots of the analysis efforts are currently being reviewed internally within the collaboration, and the aim is to have a release around September.
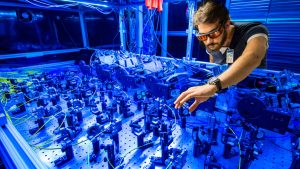
To provide context regarding the number of muons or decay positrons we measured within Runs 2 and 3, the results amount to about four times the data from Run 1. This will translate to around a factor of two improvement in the precision of the Muon g-2 experiment Run 1 measurement.
In terms of the release itself, the analysis is what we call ‘blinded’. During the whole analysis procedure, we do not know the answer. We do this to remove any potential bias that could enter. This method enables us to carry out such things as assessing our confidence in our procedures to ensure they make sense and that we are getting consistent results.
Eventually, we will reach a point where we are satisfied with the consistency checks and can agree to unblind the result. That unblinded result can then be directly compared to the previous measurements and the theory.
When will the data taking cease and what will happen at this stage?
EB: The data collection periods for this experiment normally commence in November and finish around July. During the summer, the machine undergoes a ‘shutdown’ period for maintenance of the system to make the next run possible. In particular, the magnets need to be warmed up and cooled down to ensure efficient operation next year.
This is the last round that we will do with muons and, once it is finished, we will measure the ring’s magnetic field for the next year without injecting muons inside. As Sean said, we are analysing Run 3 now, and we will then move on to analyse Runs 4, 5, and 6. This will be the largest dataset, and the analysis will take another two to three years to complete. We hope to obtain the most precise measurement of the Muon g-2 experiment.
Please note, this article will also appear in the fourteenth edition of our quarterly publication.