Dr Björn Burmann, from the University of Gothenburg’s Department of Chemistry and Molecular Biology and the Wallenberg Centre for Molecular and Translational Medicine, outlines how molecular chaperones could play a role in Parkinson’s disease.
In patients with Parkinson’s disease, there is a direct relationship between the presence of so-called ‘Lewy bodies’ in the brain and this neurodegenerative disease. Lewy bodies arise within nerve cells and consist of a medley of diverse cellular organelles and aggregated proteins, such as the protein α-synuclein (recently reviewed by H. Lashuel).1 α-synuclein is a so-called ‘intrinsically disordered protein’, characterised by the fact that these proteins do not have a fixed three-dimensional structure, but alternates between a large range of different conformations. How α-synuclein is controlled within the cell and the cellular factors that drive its structural change to insoluble aggregates remains so far unclear. Nevertheless, previous research has indicated that the propensity of α-synuclein to form these harmful aggregates dependents on a large variety of external factors, among them the interactions with molecular chaperones.
Molecular chaperones are the common name for a class of proteins that ensure that other cellular proteins fold correctly in the cellular environment to be able to fulfil their functionality. One of the key features of chaperones is that they interact with a very diverse set of clients performing different tasks.2 These jobs can be very specific depending on what kind of chaperone it is, but overall, the chaperones can be described as crucial cellular proteins that act by stabilising client proteins, preventing the folding and formation of toxic protein aggregates within cells. Some molecular chaperones have even the capability to dissolve existing protein aggregates or lead incorrectly folded proteins to the cellular systems responsible for protein clearance and degradation. Because chaperones have these important properties, together with previous research indicating that molecular chaperones have a specific way of recognising client proteins encoded in these clients, we selected several human and bacterial chaperones that all function in different ways, and tested how these chaperones interact with α-synuclein.3,4
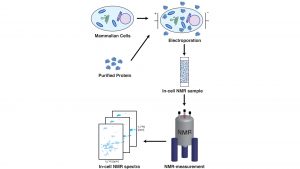
Molecular chaperones bind to α-synuclein in the cell
The technique we primarily used to investigate how α-synuclein and chaperones interact with each other is called NMR spectroscopy (nuclear magnetic resonance spectroscopy). The technique is based on the magnetic resonance of nuclear spins which is also the basis of the more commonly known medical technique magnetic resonance tomography. Briefly, with this method we are able to study at the amino-acid level the interaction between α-synuclein and chaperones by measuring spectra in the absence of binding partners, and then comparing these results with a spectrum after chaperone addition to α-synuclein in the NMR tube. The obtained results are for simplicity called intensity profiles here. In preliminary work we map which NMR signal in the spectrum originates from which amino acid in α-synuclein, we can identify exactly which amino acids are involved in the interaction between α-synuclein and a molecular chaperone.
When we started to look in detail at how chaperones interact with α-synuclein, we noticed very accurately that all of the molecular chaperones we tested recognised specific regions of α-synuclein. These regions are located in the amino-terminal portion of α-synuclein and a short region of six amino acids around the aromatic amino-acid Tyr39. Interestingly, these regions also constitute in general the most hydrophobic patches of the whole of the α-synuclein sequence.
So far, we had tested what the interactions between chaperones and α-synuclein looked like by mixing purified protein solutions in the test tube together, but of course we ultimately were aiming for if we could observe the same kind of interaction within living mammalian cells.
To achieve this, we adapted an approach pioneered by the Selenko lab in Berlin,5 by which purified isotope-labelled α-synuclein is brought into living mammalian cells by electroporation, enabling us to measure it within the cell6 (see Fig. 1). By comparing our results to previously published data showing the NMR profile of α-synuclein in living mammalian cells,5 we observed a highly similar intensity profile of α-synuclein in living cells as well as in our titration experiments.
However, the proposed model was that this profile in living cells likely arises because α-synuclein interacts with cellular membranes, in contrast that we had observed the same profile when α-synuclein interacts with chaperones. In order to clarify which of these two hypotheses would prove to be correct, we decided to develop an experimental approach able to distinguish between these two alternate scenarios.
First, we looked at the profile that arose when α-synuclein was titrated with cellular extracts devoid of any membranes. Interestingly, we observed that the obtained intensity profile of α-synuclein when titrated with chaperones is also observed when we titrated α-synuclein with cell extracts lacking any membrane vesicles, while we see a completely different type of intensity profile when we titrate down membrane vesicles. When the α-synuclein interacted with the membrane vesicles, which we observed that the protein did, a global signal reduction was observed throughout almost the entire α-synuclein, instead of only around the amino-terminus and in the vicinity of Tyr39.
Thus, our experiments showed that α-synuclein can interact with both chaperones and membranes. How stable are these types of interactions? We tested this by adding a chaperone to a solution with α-synuclein and membrane vesicles, and we then saw that the intensity profile changed back to that observed during interaction between α-synuclein and a chaperone. The opposite experiment (addition of membrane vesicles to a solution with α-synuclein and chaperones) also showed that the profile shifts to the intensity profile typical of an interaction between α-synuclein and membrane. Thus, the results clearly indicate that both chaperones and membrane vesicles compete to bind to α-synuclein, which leads to α-synuclein being in an equilibrium state between its free form, its membrane bound form and its chaperone bound form, where our experiments indicate that the two later stages cannot occur at the same time (see Fig. 2).
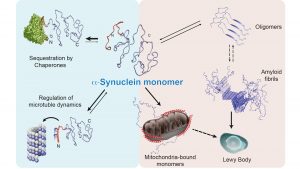
Modifications of α-synuclein may interfere with its interaction with chaperones
Using mass spectrometry, we investigated what α-synuclein mainly binds to living cells and found that α-synuclein is found bound to several different chaperones, including different variants of the most abundant molecular chaperones Hsc70 and Hsp90. We noticed that if we inhibited both Hsc70 and Hsp90, the intensity profile of α-synuclein was affected, which we did not observe if only Hsc70 was inhibited. Because cells contain several different chaperones, this indicates that Hsc70 and other chaperones have an overlapping function.
We also noticed that if we inhibited both of these chaperones, the equilibrium state of α-synuclein appears to be disturbed and more α-synuclein binds to the membrane rather than to the chaperones. Interestingly, this results in the formation of stable aggregates containing α-synuclein. We further investigated which membrane organelle that α-synuclein interacts with in this chaperone-inhibited state by means of immunofluorescence microscopy and found that α-synuclein was relocated to the mitochondria.
Finally, we took a closer look at how post-translational modifications of α-synuclein affect its interaction with chaperones. We tested several know modifications of α-synuclein: acetylation, phosphorylation, methionine oxidation as well as a mutant where we removed the amino-terminal portion of α-synuclein. Acetylation of the amino-terminal portion of α-synuclein, which is the major variant found in mammalian cells, did not adversely affect the interaction between chaperones and α-synuclein. In contrast, α-synuclein, which lacked its N-terminal portion, had a greatly reduced interaction with the chaperones, as expected. Oxidative stress and upregulation of the kinase c-Abl (Abelson tyrosine kinase) with phosphorylation of Tyr39 are known risk factors for Parkinson’s disease. We therefore investigated the effect of oxidation of Met1 and Met5, as well as different phosphorylation patterns of tyrosines in α-synuclein. We could see that oxidation had a negative effect on the interaction between α-synuclein and chaperones. For phosphorylation, we observed that the phosphorylations involving Tyr39, either individually or together with Tyr125, Tyr133 and Tyr136, had a negative effect on the interaction between α-synuclein and chaperones. In contrast, phosphorylations that did not include Tyr39 had no adverse effect on the chaperone-α-synuclein interaction.
What do our results mean?
In summary, our results indicate that α-synuclein has a distinct recognition sequence that is recognised by several different chaperones, and they also provide completely novel insights into how molecular chaperones regulate α-synuclein via this interaction. In our model, the majority of α-synuclein is bound to chaperones as the cells function normally and are healthy. Based on data on the concentration of α-synuclein and the chaperones Hsp70 and Hsp90 in nerve synapses, we estimate that approximately 90% of α-synuclein is bound to molecular chaperones effectively controlling the amount of free α-synuclein.
By contrast, when the balance between α-synuclein and chaperones is disturbed, α-synuclein interacts more extensively with cellular membranes, relocates to the mitochondria possibly starting to integrate into the mitochondrial membranes as recent studies suggest7 as well as starting to form protein aggregates. It is already known that Lewy bodies are largely composed of both α-synuclein and mitochondrial fragments, and impaired mitochondrial function has been suggested as a contributing factor behind Parkinson’s disease, which could be explained by our model.
In our experiments, we have used specific inhibitors for the Hsc70 and Hsp90 chaperones, but the most important question is of course what causes the same phenomenon naturally. In our model, both altered amounts and/or altered activity of both chaperones and α-synuclein could interfere with the equilibrium balance, but as we have shown, post-translational modifications may also affect the interaction between chaperones and α-synuclein. Previous research in the field has shown that within hereditary Parkinson’s, the ratio between α-synuclein and chaperones is impaired as well as that with aging the concentration of cellular chaperones is reduced.
Another important driver of neurodegenerative diseases is oxidative stress. This has also been shown to be associated with increased phosphorylation of Tyr39 by α-synuclein,8 which we could show in our study to have an inhibitory effect on the interaction between α-synuclein and chaperones. The kinase we used for monophosphorylation of Tyr39, so-called ‘c-Abl’, is also interesting from the perspective that upregulation of c-Abl correlates strongly with the specific phosphorylation of Tyr39 and Parkinson’s disease. This link has already been started to be exploited in the clinics, as initial studies suggested the benefits of the usage of inhibitors of c-Abl initially developed for its participation in a severe form of leukemia, acute myelogenous leukemia.9
Collectively, our recent results form a novel picture of how α-synuclein is regulated within the cellular context by molecular chaperones, and shows how a non-functioning interaction between chaperones and α-synuclein can result in α-synuclein forming aggregates as which might represent a crucial initial process leading to cellular dysfunction in neurons and subsequently to the neurodegenerative disease. In addition, the direct link to the mitochondria is highly intriguing as it becomes more and more understood that mitochondria play a central role in Parkinson’s and we have started to investigate how α-synuclein might be affected by mitochondrial proteins. In summary, we hope that our studies will lead in the future to new types of drugs or therapies being developed based on the role of chaperones in regulating α-synuclein.
Acknowledgements
B.M.B. gratefully acknowledges funding from the Swedish Research Council (Vetenskapsrådet Starting Grant 2016-04721) and the Knut och Alice Wallenberg Foundation through a Wallenberg Academy Fellowship (2016.0163) as well as through the Wallenberg Centre for Molecular and Translational Medicine, University of Gothenburg, Sweden. The Swedish NMR Centre of the University of Gothenburg is acknowledged for spectrometer time.
References
- Lashuel, H.A. (2020) ‘Do Lewy bodies contain α-synuclein fibrils? and Does it matter? A brief history and critical analysis of recent reports’. Neurobiol. Dis., 104876
- Burmann, B.M. and Hiller, S. (2015) ‘Chaperones and chaperone-substrate complexes: Dynamic playgrounds for NMR spectroscopists’. Prog. Nucl. Magn. Reson. Spectrosc., 86-87, 41–64
- Burmann, B.M., Gerez, J.A., Matečko-Burmann, I., Campioni, S., Kumari, P., Ghosh, D., Mazur, A., Aspholm, E.E., Šulskis, D., Wawrzyniuk, M. et al. (2020) ‘Regulation of α-synuclein by chaperones in mammalian cells’. Nature, 577, 127–132
- Horvath, I., Blockhuys, S. Šulskis, D., Holgersson, S., Kumar, R., Burmann, B.M. and Wittung-Stafshede, P. (2019) ‘Interaction between copper chaperone Atox1 and Parkinson’s disease protein α-Synuclein includes metal-binding sites and occurs in living cells’. ACS Chem. Neurosci., 10, 4659–4668
- Theillet, F.X., Binolfi, A., Bekei, B., Martorana, A., Rose, H.M., Stuiver, M., Verzini, S., Lorenz, D., van Rossum, M., Goldfarb, D. et al. (2016) ‘Structural disorder of monomeric α-Synuclein persists in mammalian cells’. Nature, 530, 45–50
- Matečko-Burmann, I. and Burmann, B.M. (2020) ‘Recording in-cell NMR-spectra in living mammalian cells’. Methods Mol. Biol., 2141
- Fusco, G., Chen, S.W., Williamson, P.T.F., Cascella, R., Perni, M., Jarvis, J.A., Cecchi, C., Vendruscolo, M., Chiti, F., Cremades, N. et al. (2017) ‘Structural basis of membrane disruption and cellular toxicity by α-Synuclein oligomers’. Science, 358, 1440–1443
- Mahul-Mellier, A.L., Fauvet, B., Gysbers, A., Dikiy, I., Oueslati, A., Georgeon, S., Lamontanara, A.J., Bisquertt, A., Eliezer, D., Masliah, E. et al. (2014) ‘c-Abl phosphorylates α-Synuclein and regulates its degradation: implication for α-Synuclein clearance and contribution to the pathogenesis of Parkinson’s disease’. Hum. Mol. Genet., 23, 2858–2879
- Brahmachari, S., Karuppagounder, S.S., Ge, P., Lee, S., Dawson, V.L., Dawson, T.M. and Ko, H.S. (2017) ‘c-Abl and Parkinson’s Disease: Mechanisms and therapeutic potential’. J. Park. Dis., 7, 589–601
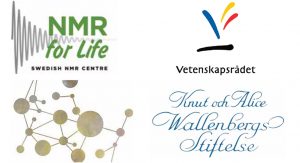
Dr Björn Burmann
Assistant Professor
Burmann Group
Department of Chemistry and
Molecular Biology
Wallenberg Centre for Molecular and Translational Medicine
University of Gothenburg
+46 31 786 3937
bjorn.marcus.burmann@gu.se
https://wcmtm.gu.se/research-groups/burmann
Please note, this article will also appear in the second edition of our new quarterly publication.