Dr Massimo Robberto, an AURA Observatory Scientist from the Space Telescope Science Institute, details the galactic journey to capture the first breathtaking James Webb Space Telescope images of the Universe.
On July 12, 2022, following a preview briefing at the White House on the previous evening, NASA, ESA and the Canadian Space Agency simultaneously released the first scientific images obtained with the James Webb Space Telescope (JWST). After 25 years of development, the James Webb Space Telescope images provide the finest pictures of the Universe ever obtained at infrared wavelengths, exciting and enchanting both the scientific community and the general public. JWST is a jewel surpassing all expectations.
The James Webb Space Telescope – an astronomical project
In the preceding six months, after the telescope launch on Christmas day 2021, scientists and engineers had been working 24/7 at the JWST Mission Operation Center, hosted by the Space Telescope Science Institute in Baltimore, to commission the observatory. These initial, essential activities followed with minimal deviations a master timeline carefully crafted and repeatedly rehearsed through the previous years. The first couple of weeks were spent deploying the telescope, so large that it had to be launched folded like origami into the voluminous fairing of an Ariane 5 rocket.
As the telescope and its suite of instruments started to cool down, approaching the operational temperature of about 40 degrees above absolute zero, the complex process of aligning the eighteen segments that compose the primary mirror took place. At first, this required “capturing” the eighteen hexagonal mirrors that, having been shaken at launch, behaved like independent telescopes looking at different points in the sky, out of focus and out of shape; at the end of this phase, we had eighteen excellent telescopes, aligned to coadd their light.
Together they delivered the most exquisite images ever obtained between one and five microns. But that was not enough. The following co-phasing stage perfectly matched the 18 mirrors next to each other. With an allowed maximum error of just a small fraction of the wavelength of visible light, their edges were flanked side-by-side to reproduce the single surface that would be ideally created by a single giant 6.5 meter diameter mirror. When this condition is achieved, physics does the ultimate magic: the light of all individual mirrors is not only coadded but also concentrated into the smallest theoretically possible (“diffraction limited’’) speckle delivered by a single 6.5m telescope. Only at this point, the actual commissioning of the four instruments could really begin.
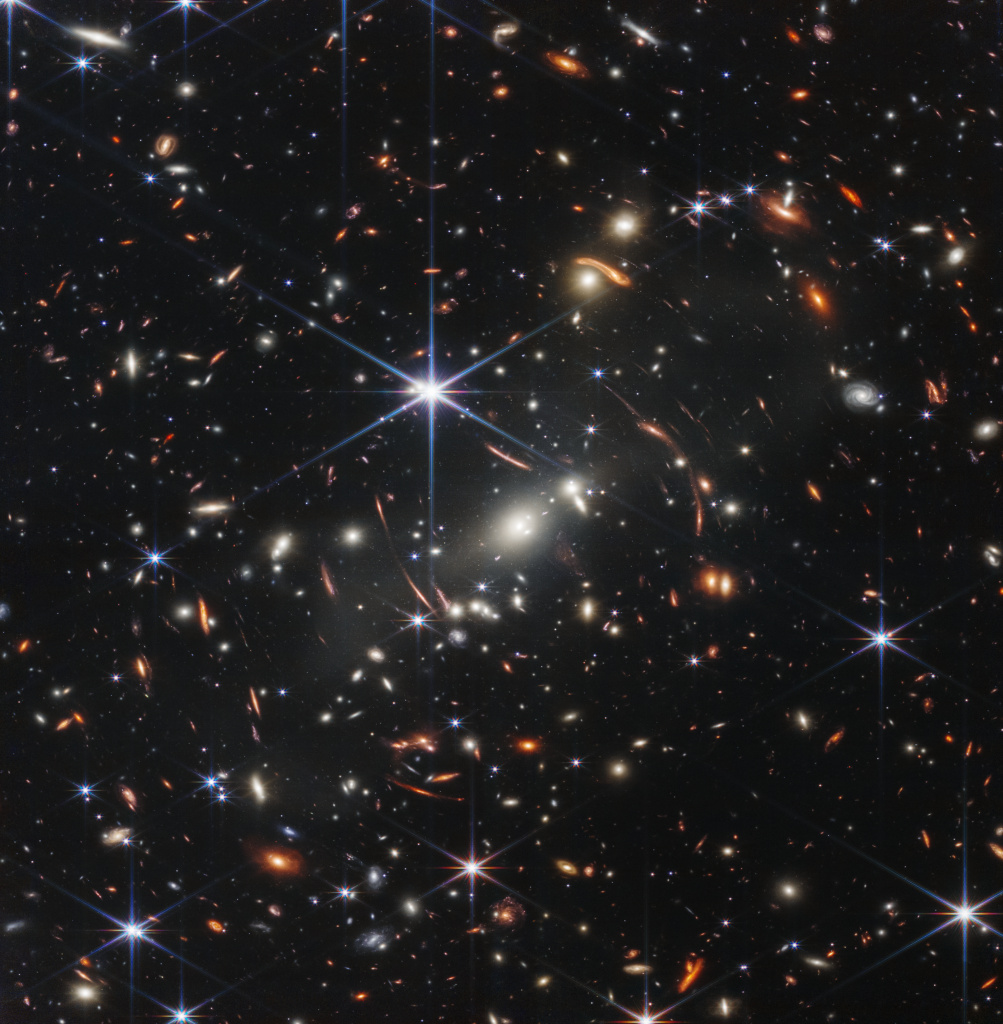
In fact, our NIRCam team had been involved in JWST commissioning almost from day one. This is because NIRCam, the Near Infrared Camera, is not only designed to be the prime, wide-field imaging instrument in the spectral region between 0.6 and five microns, but it also contains all the peculiar optical elements necessary to co-phase JWST. With the mirrors sweetly compliant to each commanded fine move, we could therefore witness the progressive improvement of the optical performance almost on a daily basis. The first snapshots obtained after capturing the mirrors already showed tens of galaxies with incredible detail. Those obtained after co-phasing, aimed at showing a single bright star with ultimate sharpness, had the sky background filled with faint galaxies. These amazing images, released by NASA to the general public, filled everybody with eager anticipation.
Then silence came. While the other instruments were coming up to speed, showcasing again hints of spectacular performance, we were all asked to concentrate on the challenging and somewhat tedious work of understanding in detail the instrument’s behaviour. At the same time, a set of initial science observations, the Early Release Observations (EROs), were planned and executed with a strict “closed-door” policy. Only a few scientists could look at the data; blackbelt experts of data processing were able to quickly create striking images mitigating all sorts of artefacts that may persist through our preliminary instrument calibration. As team lead of NIRCam, I was part of the small group managed by my colleague, Klaus Pontoppidan who convened every morning for forty days to craft this program. Our daily meetings allowed us to assess progress, inspect the images as they were produced literally overnight, explain their content to our science writers in the room, and discuss their visualisation with the graphic artists of the Office of Public Outreach. Awe and excitement filled the room. The privilege of having been among the first to see the James Webb Space Telescope images, produced by the most powerful telescope ever built, is an unthinkable gift that fills me with profound gratitude.
What will the telescope help us understand?
The ERO targets were carefully selected to be observable given the Christmas launch date and the pointing constraints of JWST, plus of course, they were expected to be quite spectacular. JWST has seventeen observing modes, surpassing by far the already remarkable versatility of its predecessor, the Hubble Space Telescope; the EROs were intended to showcase a good number of them. While the plan was to release images and spectra for four targets, nine were observed and five were finally down-selected, the others to be released in the following weeks. They are a cluster of galaxies (SMACS 0723), a star-forming region (NGC3324), a nearby group of interacting galaxies (Stephan’s Quintet), a dying star with its planetary nebula (Southern Ring Nebula) and a transiting exoplanet (WASP-96b). I will briefly introduce the first two of them.
The galaxy cluster SMACS 0723, a rather inconspicuous system when seen with the HST, shows in glorious splendour the effect of gravitational lensing. The cluster of foreground galaxies with whitish, rather ordinary colours lies in the foreground. Its light was emitted 4.6 billion years ago when the Universe was already 9.2 billion years old. Sort of close to us! Being in the foreground, it is projected on a background of remote, red galaxies, and those are the interesting ones. Their light left when the Universe was much younger, and to reach us had to find its way across the cluster, a space filled by the gravitational field of the cluster. As Einstein predicted, gravity curves space-time, deflecting the light of those distant objects. We may therefore miss the rays that were originally directed to us, but other rays sent toward other directions are bent just by the right amount: gravity acts like a lens.
The net result is that remote galaxies can appear multiple times, stretched and warped in long filaments that trace the gravitational field. Most importantly, the lens effect can magnify the size of the distant objects and enhance their brightness, providing us in practice with a second “natural” telescope. Our curiosity was immediately captured by the faintest, very red, almost point-like sources, most probably quite primordial. Immediately, we pointed to this region a different JWST instrument, NIRSPec, to obtain their spectra. They showed with unprecedented clarity the signatures of remote galaxies, allowing us to easily determine their distance. For one source, the time travel is 13.1 billion years, i.e. the light was emitted when the Universe was just 700 million years old. Not yet the absolute record, as with Hubble we managed to reach a few more primordial galaxies, but the easiness with which those incredible spectra have been obtained is stunning and foretells revolutionary discoveries.
The Carina Nebula is a complex of star-forming regions in our Galaxy, just 7,600 light-years away. It is well studied, hosting a large number of young, giant stars, including one of the most exotic celestial sources, Eta Carinae, soon to explode as a supernova. JWST was pointed away from the central regions at the edges of a flanking cavity. The spectacular image obtained with NIRCam shows the interface between the empty cavity carved by the violent ionising radiation produced by the most massive stars (not visible in the field) and the rugged structure of the molecular cloud. We called it “Cosmic Cliffs”, as it looks like the ultimate horizon between a ridge of tormented mountainous terrain and a serene night sky dotted with stars. In fact, the entire field is filled with tenuous veils, heated material evaporating from the cloud’s surface.
The hilly landscape displays the turbulent status of molecular clouds and a close inspection reveals the rich phenomenology associated with star formation: pillars are formed when clumps of dense gas pose higher resistance to the ionising radiation. Within the dense cusps, new stars may form. Strangely, they may soon contribute to the destruction of their own surroundings, ejecting material as jets that produce shock waves. Shocks travel supersonically and can give a final kick to a nearly unstable cloud in the vicinity triggering new star formation or just inject turbulence in the medium, further dissipating the cloud. Stirred and shaken, the cocktail leading to star formation is one of the most complex natural phenomena and we definitely count on JWST to help us answer our many open questions.
Today we celebrate the James Webb Space Telescope images as a triumph of technology. We can regard it as a tribute to what we can do when we work together, driven ultimately by our curiosity, fulfilling our drive to understand the cosmos, its beauty, and our place in it.