Dr Muriel Fallot, Assistant Professor at the University of Nantes (SUBATECH Laboratory) performs her work using the Total Absorption Gamma-ray Spectroscopy technique to study nuclear beta decay.
Nuclear beta decay is a simple process that is sensitive to the structure of the nucleus. Understanding this process impacts not only the fundamental studies of the weak interaction, but also nuclear astrophysics processes, reactor technology, and particle physics.
The focus of our fundamental studies is to fully understand the nuclear beta decay process in nuclei far from stability, which is carried out using the Total Absorption Gamma-ray Spectroscopy (TAGS) technique.1
The TAGS technique is the best suited to experimentally access the beta intensity, which provides an insight into the nuclear structure of exotic nuclei and new constraints on theoretical models. The TAGS method has been employed successfully by the groups involved in this collaboration in several experiments.2 This technique allows one to overcome the systematic error known as Pandemonium effect,3 which affects high-resolution data coming from experiments with HPGe detectors.
Due to the low efficiency of HPGe detectors, high-energy gamma-rays may be missed, leading to a wrong determination of the beta intensity probabilities obtained via gamma-intensity balance. The access to very short half-life nuclei is challenging, since the TAGS method, with existing detectors, requires a minimal knowledge of the structure of the nucleus under study.
The measurements performed with the TAGS technique provide excellent tests of the calculations of the astrophysical rapid neutron capture process, called r-process, responsible for the production of around half of the elements heavier than iron. During the last decade, our collaboration has an ambitious physics programme, allowing the acquisition of new fundamental knowledge in nuclear structure that are far from stable, this knowledge is essential when testing nuclear structures and understanding the models used in nucleosynthesis calculations and has a broad scientific impact on nuclear physics applications.
The kilonova, observed in association with the GW170817 gravitational waves, gave direct evidence that the r-process takes place at least in neutron star mergers.4 Several sensitivity studies indicate that the properties of many nuclei, such as masses, beta-decay half-lives, Pn values, and neutron-capture rates have a particularly large impact on the r-process calculations,5 which require theoretical predictions for nuclei too exotic to be measured.
The measurements performed with the TAGS technique provide excellent tests of these calculations. During the last decade, our collaboration has collected evidence that gamma emission from neutron-unbound states, populated in the beta decay, can be much larger than anticipated.6 Using this information to control neutron capture cross-sections for very neutron-rich nuclei far from stability, which is otherwise obtained from theoretical estimates, has been proposed.6 We were also among the first to propose the study of pygmy modes through beta decay.7 In very neutron-rich nuclei, the neutron excess could constitute a neutron-skin around a core, composed of the remaining nucleons. The excitation of the neutron-skin with respect to the rest of the nucleus is a low-lying collective excitation called pygmy resonance. The presence of these low-lying collective modes in very neutron-rich nuclei strongly modifies the r-process path.8 Due to its high efficiency to high-energy gamma-rays, the TAGS technique could be a unique way to experimentally access information relevant for the r-process, where direct measurements are not possible yet.
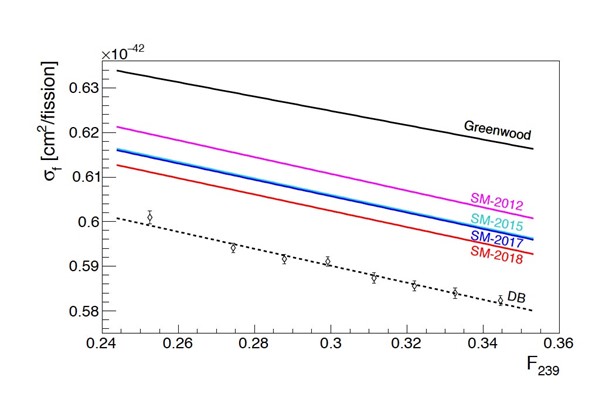
A major concern in neutrino physics has been the better determination of the oscillation parameter θ13 with large detectors placed close to nuclear power plants.9,10,11 The detection of antineutrinos has also been proposed as a potential tool for reactor monitoring, as the measured properties of the antineutrinos reflect the reactor fuel composition.12 In this context, a re-evaluation of the reactor antineutrino energy spectrum,13,14 based on the conversion of integral beta energy spectra measured by K. Schreckenbach and co-workers at the high-flux reactor of the Institut Laue-Langevin, Grenoble, France, led to the reactor antineutrino anomaly: (RAA) – a deficit of about 6% between the theoretical prediction and the neutrino experiments at short baselines. It also led to the shape anomaly: a distortion of the measured energy spectra by the large reactor neutrino experiments with respect to the converted spectra in the 5 to 7 MeV antineutrino energy region.16
While it could have been triggered by an underestimation of the systematic errors of converted spectra, exciting new areas of physics could arise from these anomalies, such as the existence of light sterile neutrinos.17 Clearly, nuclear physics could help to solve this particle physics puzzle. The antineutrino spectrum can be calculated using the information contained in nuclear decay databases of the fission products (summation method, SM).13 However, it was shown that some of this data suffers from the Pandemonium effect.18 The situation is similar to that found in the evaluation of the reactor decay heat.20,21
The TAGS collaboration has carried out two experimental campaigns at the JYFLTRAP of Jyväskylä, Finland, measuring a large set of data to improve the quality of the predictions for antineutrino spectra and reactor decay heat. 22-27 The antineutrino spectrum and flux obtained,28 compared to the Daya Bay experiments,18 shows the best agreement in shape in the energy range 2 to 5 MeV and in flux obtained so far with a model. The flux deficit observed by Daya Bay, in regards of the SM, is reduced to 1.9%, leaving little room for the RAA, provided that the correction of more Pandemonium data should reduce this discrepancy (see Fig. 1). The shape anomaly remains unexplained. Going forward, efforts should be focused on nuclei with data still suffering from the Pandemonium effect, contributing mostly to the energy range above 4.5 MeV. The challenge to obtain reliable calculations of the antineutrino spectra over the full energy range will be to perform TAGS measurements on these nuclei which lie further from stability than previously.
As quoted above, the Pandemonium effect impacts the summation calculation of another quantity of interest for reactor safety, the decay heat (DH).20 DH is the energy released by the nuclear fuel, following the shutdown of the chain reaction in a reactor. It is essentially made up of radioactive (β, γ, and α radiations) decays of the fission products (FP) and actinides.
On average, it represents a residual power of 6 to 12% of the nominal power of the reactor just after its shutdown. These numbers are huge and motivate the further understanding of the DH, for both the evaluation of the reactor safety, and various economic aspects of nuclear power generation. Important discrepancies have been observed between summation calculations which used the information stored in nuclear databases held worldwide and calculations from benchmark experiments.20,21 Recently, an international effort has established a priority list of TAGS measurements. The long-standing electromagnetic component of the DH puzzle after the fission of 239Pu was partially solved in 2010 following the first TAGS campaign in Jyväskylä of the Valencian group.21 This crucial result stimulated the prolonging of the experimental effort with our second TAS campaign in Jyväskylä. Since then, the TAGS measurements of 12 decays were performed and published in Journal of Physics.2 We have measured 17 nuclei out of the 37 nuclides identified by the WPEC-25 (NEA working group) for TAGS measurements to improve the predictions of the decay heat in conventional reactors.
The access to more exotic nuclei with the TAGS technique is challenging; current limitations of the method could be lifted with the realisation of an advanced Total Absorption Spectrometer (TAS) with improved efficiency and energy resolution.
The TAGS experiments quoted above have been proposed by the teams of l’Instituto de Fisica Corpuscular (IFIC), Spain, Subatech (CNRS-in2p3, Univ. Of Nantes, IMTA), France, and the University of Surrey, UK in collaboration with international collaborators.
References
- V. Guadilla et al., Nucl. Instr. Meth. A910, 79-89 (2018).
- A. Algora, J.-L. Tain, B. Rubio, M. Fallot, W. Gelletly, Eur. Phys. J. A (2020).
- C. Hardy et al., Phys. Lett. 71 B, 307 (1977).
- B. P. Abbott et al., Phys. Rev. Lett. 119, 16 (2017).
- M. R. Mumpower et al., PPNP 86, 86 (2016).
- L. Taìn et al., Phys. Rev. Lett. 115, 062502 (2015).
- Workshop on “Collective Mode Study through Beta-Decay Measurements”. Subatech, Nantes ; 19-20 January 2015. https://indico.cern.ch/event/354566/.
- S. Goriely, Physics Letters B 436, 10 (1998).
- Y. Abe et al., Phys. Rev. Lett. 108, 131801 (2012).
- P. An et al., Phys. Rev. Lett. 108, 171803 (2012).
- K. Ahn et al., Phys. Rev. Lett. 108, 191802 (2012).
- A. Mikaelian, Proc. Int. Conf. Neutrino-77, v.2, 383 (1977).
- A. Mueller et al., Phys. Rev. C 83, 054615 (2011).
- P. Huber, Phys. Rev. C84, 024617 (2011).
- A. Hahn et al., Phys. Lett. B 218, 365 (1989) and references therein.
- Double Chooz and Reno presentations at Neutrino 2014 conference, http://neutrino2014.bu.edu/, Daya Bay presentation at ICHEP 2014 conference, http://ichep2014.es/.
- G. Mention et al., Phys. Rev. D 83, 073006 (2011).
- P. An et al. PRL 118, 251801 (2017). APS Viewpoint: M. Fallot, Physics 10, 66 (2017).
- M. Fallot, S. Cormon, M. Estienne et al., Phys. Rev. Lett. 109, 202504 (2012).
- T. Yoshida et al., OECD/ NEA Working Party for International Evaluation Cooperation, 1425 , 25 (2007). Nuclear Science NEA/ WPEC-25 (2007).
- A. Algora et al., Phys. Rev. Lett. 105, 202501 (2010).
- A. Zakari-Issoufou et al., Phys. Rev. Lett. 115, 102503 (2015).
- E. Valencia et al., Phys. Rev. C 95, 024320 (2017).
- S. Rice et al., Phys. Rev. C 96, 014320 (2017).
- V. Guadilla et al., Phys. Rev. Lett. 122, (2019) 042502.
- V. Guadilla et al., Phys. Rev. C 100 (2019) 024311.
- V. Guadilla et al., Phys. Rev. C, accepted 24 September 2019.
- M. Estienne et al., Phys. Rev. Lett. 123, 022502 (2019).