Anne Dutrey, from the Laboratory of Astrophysics of Bordeaux at Université de Bordeaux – CNRS, outlines new research utilising ALMA to search for planet formation in disks orbiting young stars
Understanding planet formation is a major challenge of modern astrophysics. Planets form in molecular clouds from the disk rotating around the newly born star, a residual of gas and dust left over after the star formation. Observing such protoplanetary disks remains difficult. Disks are small, with typical sizes of ~50-200 astronomical units (au) or 0.4-1.6’’ at 120 parsecs (pc), the distance of the nearest star forming regions, and cold (temperatures of about 10-30 K at 50 au), thus essentially radiating at long wavelengths in the millimetre/submillimetre (mm/submm) domain. It is only since 1990 that large mm/submm interferometers such as the IRAM array (French Alps) have been able to provide the first resolved images of these objects.
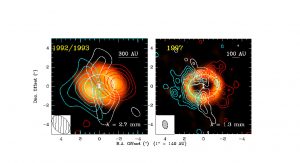
Fig. 1 shows one of the most spectacular disk observations of this period. The dust and gas disk is in Keplerian orbit around the low-mass (1.3 Msun) binary star GG Tau A. The observed central cavity results from tides due to gravitational interactions between the binary, which orbits around its mass centre, and the outer disk.
Binary stars need to be studied because they are common in our Galaxy (about 50% of Main-Sequence stars) and potentially represent an important reservoir of planetary systems, despite a complex, gravitationally disturbed, environment. The KEPLER mission, for instance, has revealed that planets can exist in both circumbinary and circumstellar orbits.
ALMA
With the advent of the Atacama Large Millimeter/submillimeter Array (ALMA) in Chile, in 2012, the jump in linear resolution from 200-300 (two to three times the size of the inner solar system) to 10-50 au makes the observations of nascent planetary systems feasible. ALMA observations of disks have revealed gaps and spirals that may result from the formation of unseen embedded planets. With my team and collaborators, instead of doing large surveys, we decided to use ALMA to accurately determine physical, chemical, and dynamical properties of some proto-typical disks to constrain planet formation scenarios.
GG Tau A was our prime target. Our main goal was to determine how the reservoir of matter in the outer disk is accreted onto the central stars. In Dutrey et al 2014 (Nature, 514), we showed that accretion proceeds, as expected from theory, through filaments of gas and dust falling down from the outer disk onto the inner disks. Our CO map also revealed a hot spot located at the outer edge of the dense ring (in which 80% of the disk mass resides), which could be indirect evidence of an embedded accreting planet.
In fact, a planet is too small to be observed in the mm/submm domain, but the gas accreted from its close environment is heated by accretion shocks making it indirectly visible. Such an object can naturally explain why the ring remains so dense: the matter is gravitationally confined by interactions with the binary on the inner side and with the young (Neptune-like) planet on the outer one.
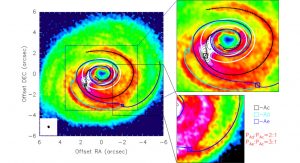
Deeper ALMA images of CO J=2-1 (Phuong et al 2020) revealed several spirals in the outer disk, the most prominent one originating from the hot spot, as expected if gravitational disk-planet interactions occur there. Moreover, two other Neptune-like planets may be necessary to explain less bright spirals (see Fig. 2). For the first time, gas spirals have been observed around multiple young, low-mass stars, confirming the ability of these systems to form planets even far away from the host stars.
AB Aurigae
Our second target is AB Aurigae, a Herbig Ae star of mass 2.4Msun. It exhibits a remnant extended envelope and a large disk with a central cavity (radius 70-80 au) of unknown origin: the star being single, this suggests the presence a young planetary system. Extended large spirals were also reported from the images of dust scattering the stellar light at the disk surface in the Near Infra-Red (NIR). Our large-scale CO images from the IRAM array (Tang et al., 2012, AA, 547) showed spirals connecting the envelope to the disk. Surprisingly, their kinematics (that can only be addressed by spectroscopy) are in counter-rotation compared to the CO disk rotation. We interpreted this as accretion flows falling onto the disk at high altitudes, the spirals appearing counter-rotating by projection effects.
At smaller scales, ALMA observations of 12CO J=2-1 at 0.07’’ or 10 au (Tang et al., 2017) revealed two gas spirals inside the disk cavity. As for GG Tau A, these inner spirals may result from gravitational interactions due to one or two planets orbiting inside the cavity. The CO spirals are also seen in NIR dust observations (Boccaletti et al. 2020, AA, 637L) in H-Band polarised emission with VLT/SPHERE. The higher angular resolution of SPHERE observations even completes the spiral shape down to about 16 au, revealing a bright twist at radius about 35 au (see Fig. 3, right).
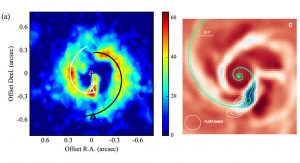
Gravitational interactions of a proto-planet with its environment are expected to produce such a twist that connects the inner and outer spirals at the location of the accreting planet. Comparing the positions of the CO emission peak in ALMA (the hot spot) and of the twist in the SPHERE images, there is a rotation of 14±1º in 4yr (the difference in time between the two data sets). This is consistent with the orbital motion of a planet at 35 au from this 2.4 Msun star. This is the first time that such orbital motion of a protoplanet is observed.
SPHERE observations also suggest the existence of a second planet at the outer edge of the cavity. The large cavity cannot be simply explained by two objects but requires the presence of several massive, still unseen, bodies. Therefore, we are just starting to unveil a nascent planetary system.
To go beyond
Studying gaps and spirals is only one aspect of planet formation. It requires observing disks seen almost face-on and the resulting opacity of the medium along the line of sight precludes any direct observation of the mid-plane where planets remain. In the case of GG Tau A, we demonstrate (Phuong et al., 2020) that most of the 12CO J=2-1 emission arises from 2.5 scale-heights above the mid-plane. This limitation can be overcome by observing edge-on disks that provide a direct view of the dust and gas radial and vertical distributions.
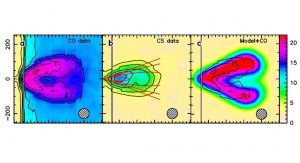
In our team, we study the Flying Saucer, an edge-on disk orbiting around a 0.7 Msun in the Rho Oph cloud. Thanks to ALMA, this disk is the first where dust settling (large particles falling by gravity onto the mid-plane where they can grow and form planetary embryos) is reported (Guilloteau et al., 2016, AA 586L). We also achieved the first CO and CS tomography (see Fig. 4). The CS molecule is characteristic of dense medium and seen around the mid-plane. CO, on the contrary, is easy to observe and characterises the whole gas distribution. Moreover, the CO J=2-1 line being optically thick and thermalised, its tomography is a direct measurement of the kinetic (gas) temperature. The deficit of molecules (either CS or CO) near most of the mid-plane is due to very low temperatures, the molecules being frozen on grain surfaces. While we wait for new ALMA observations, we are working on gas-grain chemical models to explain molecule distributions.
While the gas is the main driver of dynamics, dust leads to the formation of planetary embryos and is a catalyst for chemical reactions. Investigating the first steps of planet formation thus requires a comprehensive picture of gas and dust properties as a whole.
Anne Dutrey
Directrice de Recherche au CNRS
Laboratory of Astrophysics of Bordeaux
Université de Bordeaux – CNRS
+33 (0)5 40 00 21 84
anne.dutrey@u-bordeaux.fr
Tweet @LabAstroBord
https://astrophy.u-bordeaux.fr/
Please note, this article will also appear in the third edition of our new quarterly publication.