Professor Dr P Martin Sander of the University Bonn’s Paleontology Department takes us on the fascinating journey that forged human evolution and explains how studying dinosaurs can not only enhance our understanding of human anatomy but also inform modern medical science.
How did we come to be? The age-old question that all of humanity has, at some point, pondered. Why are we relatively diminutive compared to some of the animal kingdom, walk upright, and why can we not fly? As primates, tracing back and studying our mammalian lineages would certainly be the optimal method to further our knowledge of human anatomy, as what do we have in common with dinosaurs? A lot more than you think.
Palaeontology can provide valuable insights into the medical field, most notably human anatomy, as studying fossils can illuminate a lot about modern animals and humans. Between 250 and 200 million years ago was the Triassic period – a time that began with the biggest mass extinction event on Earth and the most pivotal time in history for our modern world – as mostly anything still around today or that existed during the dinosaur times originated in the Triassic.
Our mammalian ancestors narrowly made it into the Triassic, emerging 210 million years ago, when monstrous, 600-kilogram carnivorous dinosaurs roamed the landscape. So how did we survive this predatory era? To understand the features we acquired through evolution to survive such a harsh predation pressure, we need to look at the structure of dinosaurs that shaped our human anatomy.
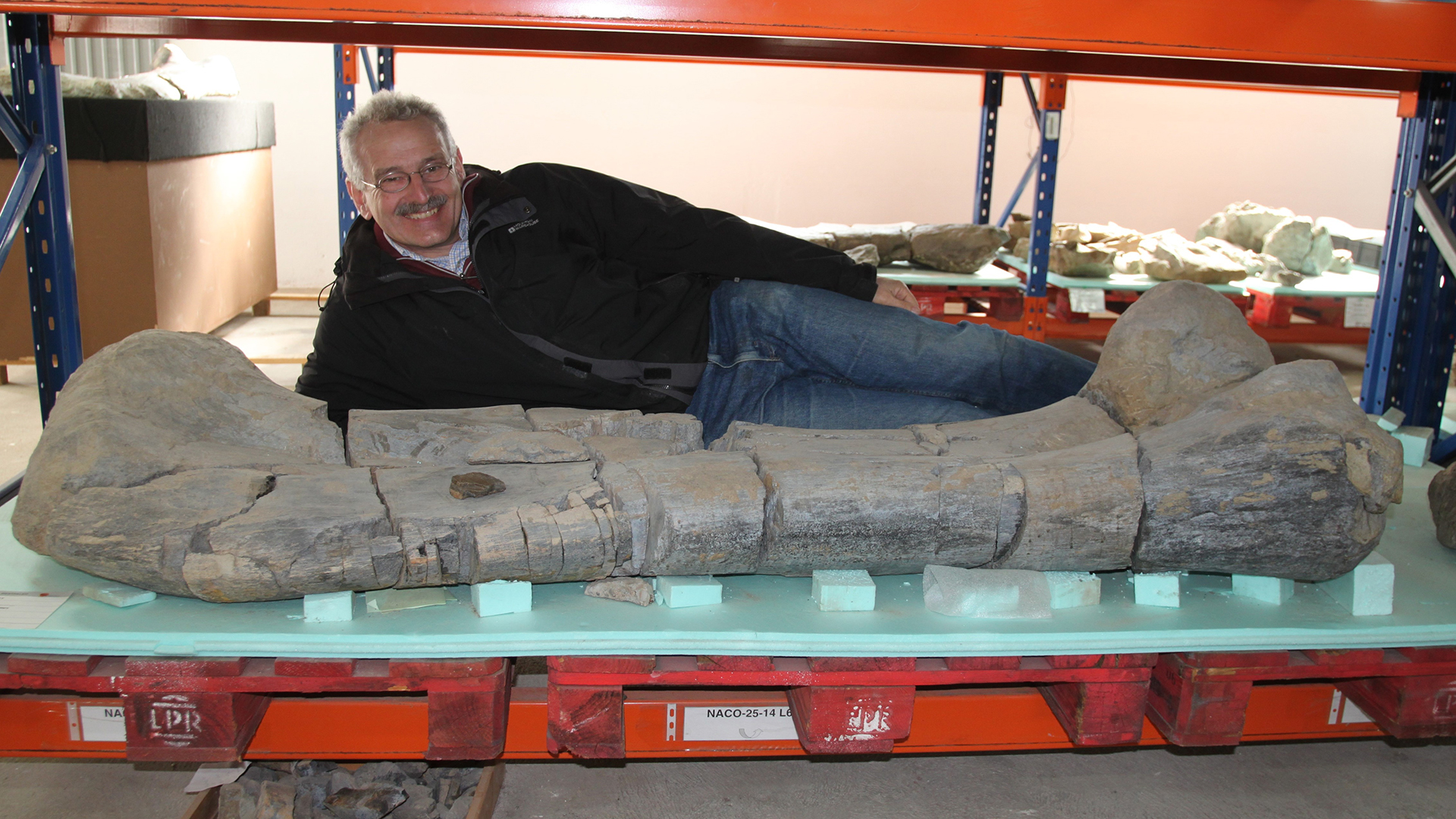
Why did dinosaurs get so big?
The obvious characteristic that sets us apart from dinosaurs is their colossal size, but why did they become so extraordinarily large, dwarfing the animals we see today? Research Unit 533 at the University of Bonn set out to answer this question, analysing an especially large type of dinosaur, even by dinosaur standards, known as sauropod dinosaurs. These are the dinosaurs with the extremely long neck.
In biology, animals are measured by mass. Typically, a big sauropod is 80 metric tonnes, whereas a very big elephant is eight metric tonnes, roughly a ten-fold increase in body mass. Some dinosaurs were a bit larger, but on average, all dinosaurs were larger than mammals. The team asked a fundamental question: is there a biological or geological explanation for this?
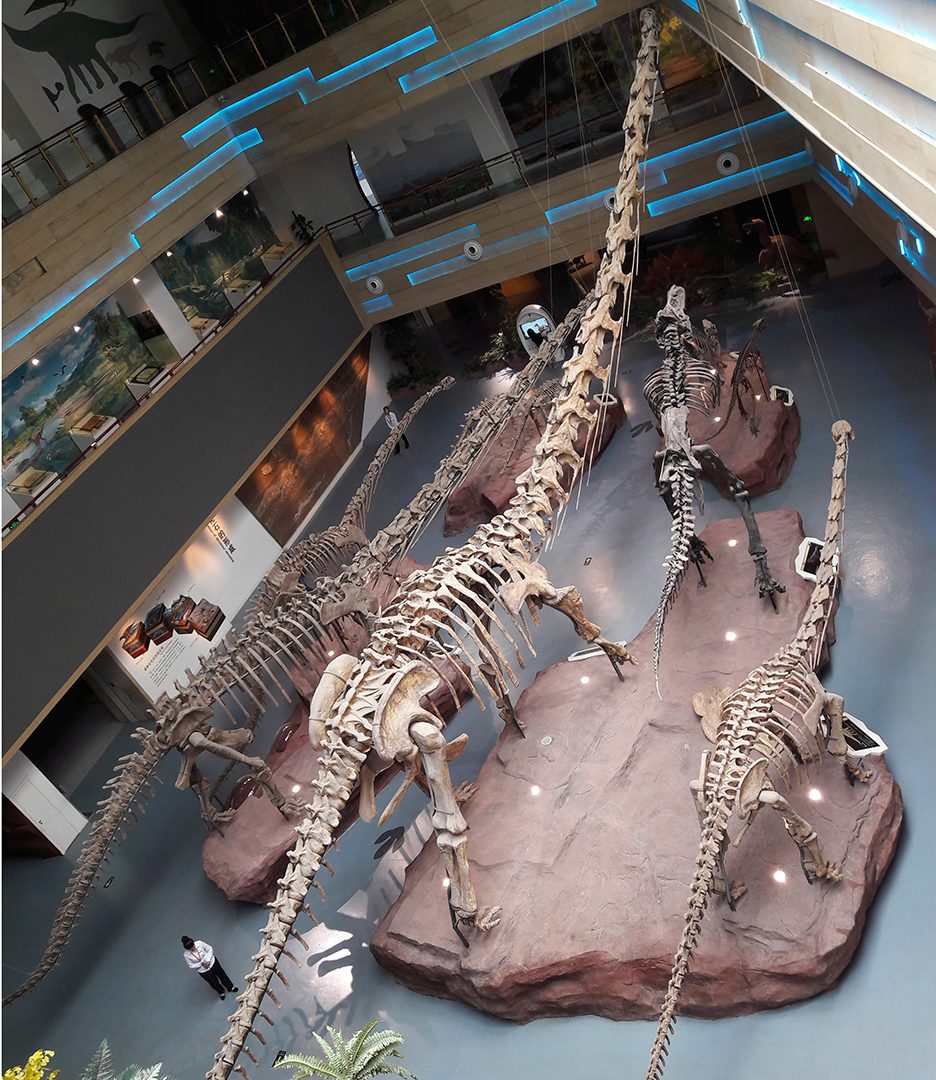
To explore this, they used the nature vs nurture argument. Nurture would represent climate change; for example, in the age of dinosaurs, the Earth was so warm that it was easier to become large, and nature would be the biology of the animals. The team rapidly concluded it had to be the biology by tracking oxygen content of the atmosphere.
During the beginning of the period of dinosaurs, the planet’s oxygen content was around 15% – which would be equivalent to being 2,500 metres above sea level. However, by tracking parameters, such as oxygen content or global temperatures, they identified that body mass or body size does not correlate to these conditions. Low or high oxygen content did not influence body mass, meaning sauropod gigantism was due to their biology.
Optimisation through evolution
A common misconception about evolution is that animals become perfectly optimised. This is not the case. It is only locally optimised. Evolution does optimise, but it does not always find the optimal solution. For example, when improving a car, you work with what you have. You can improve the components, but you are only enhancing the base design. The team discovered that gigantism is related to a complex interplay of primitive features and evolutionary novelty. In technology, you have a similar effect. Important novelties would include the aluminium rim, air-filled tyres, and diesel engines. Evolution is the same, and this interplay of primitive inherited features and evolutionary novelty caused this ten-times larger body size.
Evolutionary novelties
Notable primitive features of sauropods include having many small offspring by laying eggs and not chewing their food. Evolutionary novelties were a high metabolic rate or warm-bloodedness and having a bird lung, but all four are essential prerequisites of gigantism. Contrastingly, we mammals have few, larger offspring, chew our food, and do not have a bird lung. But we also are warm-blooded.
So how do these evolutionary novelties precipitate gigantism? Firstly, the bird lung is advantageous in several ways as it can efficiently take up oxygen. That is why birds can fly over the Himalayas with no problem, which means the cost of breathing is less, so you have more energy to do other things.
Birds also have a system in their bones known as pneumaticity, where they can bring air into their bones, a trait that sauropods share—replacing bone marrow with air results in a lightweight skeleton. This means that you can have a super long neck and, because you do not need to chew, can have a small head. These characteristics meant that sauropods could gather energy more efficiently and subsequently become larger.
So why does chewing limit mammalian body size? This can be explained by the concept of scaling.
The inhibiting nature of chewing
Scaling is the concept that with increasing size, parameters must change. For example, volume increases with the third power whereas surface increases with the second power, meaning an animal that is twice the length has four times the surface and eight times the volume.
When we chew food, that is energy input for the body. However, chewing performance only increases with the second power because chewing is determined by two surface areas – the grinding surface of your teeth and muscle power. This is why elephants can’t gallop, and humans cannot jump five metres high because our muscle power is always lagging behind our body mass.
Therefore, for mammals’ size to dramatically increase, they would need to chew significantly more. For example, to maintain its weight of five tonnes, an elephant needs to chew around 18 hours a day. To increase their mass to 50 tonnes, elephants would need to increase this tenfold, which is just not possible. So – a 50-tonne elephant would only consist of a giant head that is chewing and nothing else to sustain itself.
How does this help us to understand human anatomy? Understanding our evolutionary limits illustrates why we developed certain mammalian features. In evolution, life will go where it can. If a larger body size is possible, then it will evolve in this direction, and then it will reach its limit. Have you ever wondered why the tallest trees in different parts of the world are always around 110 metres? That seems to be a limit, and of course, the mammal limit is lower than the dinosaur limit.
What makes us mammalian is the result of competition and wanting to evade the dinosaurs. We did not want to be eaten. In evolution, different lineages evolve, compete, and then eventually, one becomes extinct. Our mammalian lineage survived, but how?
Life as an early mammal
Our ancestors’ unforgiving, competitive environment was the instrumental factor as to why we evolved the features of the human anatomy we see today. Most mammals have poor eyesight, with most unable to see colour, whereas birds have highly-precise vision allowing many of them to view the ultraviolet spectrum of colour. Us primates evolved the ability to see colour, with the common reasoning being that this was essential for seeing if the fruit we were eating was ripe or not.
All mammals are warm-blooded, but smaller mammals, like us, also evolved insulation (hair). Larger animals, such as elephants, struggle to get rid of heat, which is why they are hairless. Another defining characteristic of humans is our acute hearing due to our ears being extremely complex. A primitive land animal’s ear consists of a simple, single ear bone; in contrast, the human ear comprises of three.
We evolved these distinctive features because mammals adopted a nocturnal, burrowing lifestyle to avoid being eaten. It is cold at night, so you need insulation if you are small, and if you want to burrow to get out of the way of dinosaurs and sleep in a burrow, you need to be small. These nocturnal and burrowing tactics explain why we are warm-blooded, have insulation, and cannot see well but hear well.
Our teeth are another feature exclusive to mammals, not that we have them, of course, but that we only replace them once. The lifespan of a Tyrannosaurus was between 30 and 50 years, and during this time, they replaced each of their teeth every two and a half years, meaning dentists would be out of a job during the Mesozoic, the Age of Dinosaurs.
In contrast, most mammals only replace their teeth once in their lifetime, but why? The reason we reduced our tooth generations ties in with what makes us mammalian – we suckle our young. Reducing tooth generation is optimal for breastfeeding – if there is no requirement for this type of energy transfer from parent to offspring, then there is no need to reduce tooth generation. This reduction in tooth generation has only happened once in early mammals.
We would need to have another extinction event and then look 500 million years into the future to see if this reduction in tooth generations evolved again because many things evolve repeatedly, especially distinctive features. An example of this is powered flight, which evolved three times, in pterosaurs or flying reptiles, birds, and bats.
Application in medical science
So, what can palaeontology teach us about human anatomy? Perhaps one of the most exciting discoveries of the 21st century was achieved by Svante Pääbo, who earned the Nobel Prize in Physiology or Medicine for his discoveries this year. Pääbo sequenced the Neanderthal genome and paved the way for studying the DNA of extinct organisms.
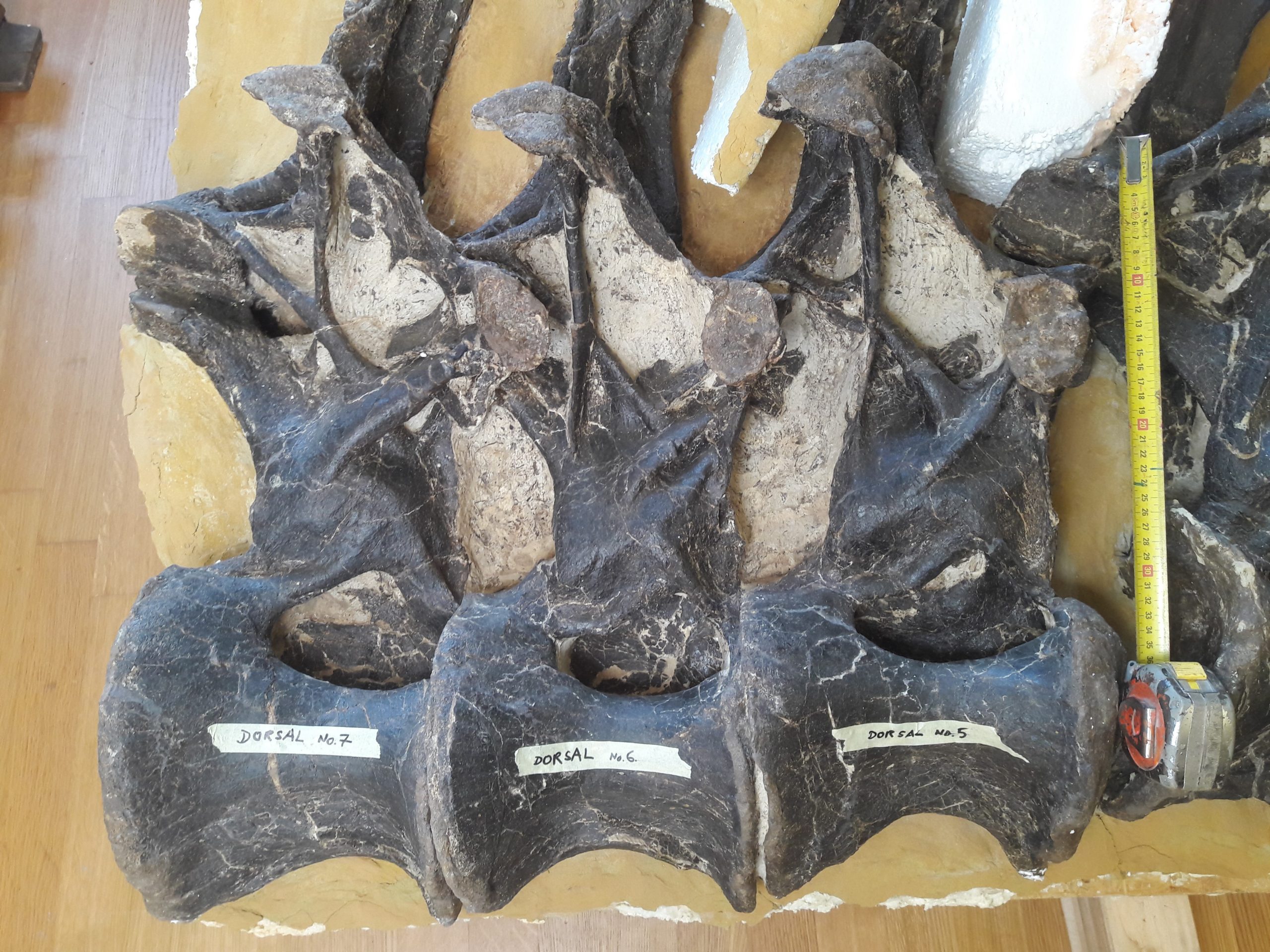
Preserved genomes only go back around two million years, but this was enough time to show that we have basically three or four species of humans. Then there is us and our next of kin – Neanderthals – who would be considered human, but they are their own species. Gene exchange, or mating, occurred between humans and Neanderthals, resulting in Caucasians today having around 2% of Neanderthal genes. This knowledge can be directly applied to the medical field regarding drug development, as knowing what these genetic features do helps us understand how they impact pathologies.
People commonly have a misleading understanding of evolution being optimised in the medical field. An example of this is our intervertebral disks, which can result in what is known as a slipped disk in our necks or backs, or dislocation in the joint. Many of us have problems with intervertebral discs, so why did we not evolve a better type of joint? The reason relates to the earlier point that evolution is only locally optimised, meaning if you start with an intervertebral disc, you are stuck with it.
If we analyse other animals, we can find a type of joint that would be more optimal – the ball and socket joint – seen in the necks of birds, camels, and also in sauropod dinosaurs. Humans do have ball and socket joints in our shoulders, but the reason we did not evolve this handy trait in our necks is that our ability to do so went extinct many millions of years ago in the lineage leading to mammals. This insight was gained from studying thin slices of bone cut from fossil vertebra under the microscope.
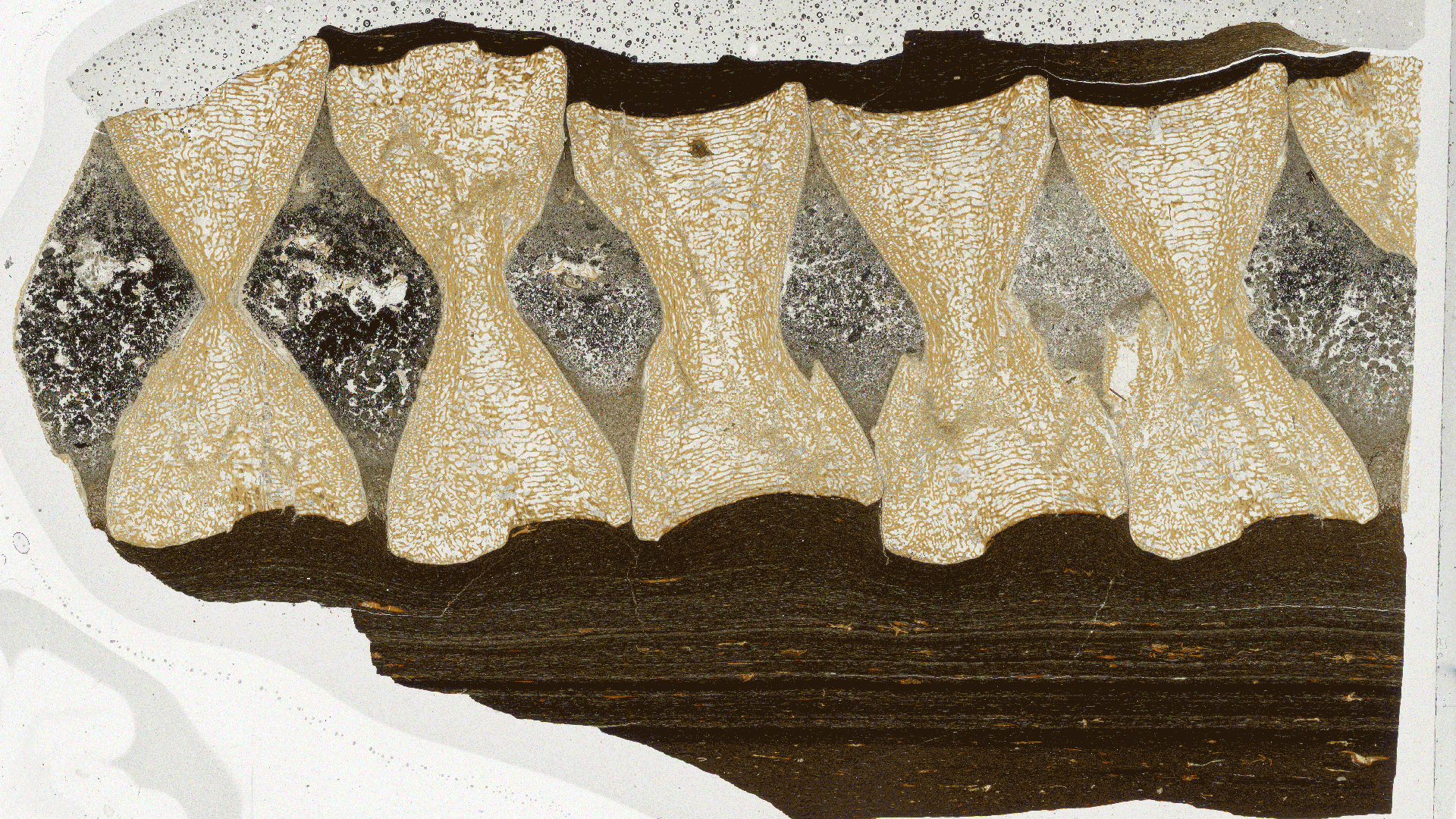
We see this pattern often. Certain things just things disappear off the face of the Earth. An interesting example of this is that when animals first crawled on land, we experimented with a number of fingers. Today, most mammals, lizards, and amphibians have five fingers, so it was a huge surprise around two decades ago when palaeontologists discovered that the early land animals had six, seven, and even eight fingers. Eventually, the developmental pathway for these genes went extinct, leaving the five fingers we see today.
So, what is the medical application for this? There was pathology in Europe in the early 60s that led to some children being born with fewer fingers. This was caused due to women being prescribed a sleeping pill called thalidomide that led to their children being born with malformed limbs. This pathology can be compared to that of the tyrannosaur.
The tyrannosaur had proverbial short arms comprised of two interesting patterns: their upper arm bone was very small, and they only had two or sometimes three fingers. This same pattern is observed in these children, as their limb development is truncated at an early stage. Limbs develop from close to the body to further away, meaning that thalidomide led to a reduced number of fingers.
Although the tyrannosaur never suffered from a sprained wrist and dinosaur’s bird lungs meant they never developed asthma, despite our obvious differences, palaeontology can provide crucial insights into human anatomy. By looking at our inner dinosaur, we do not just understand where we came from but also the reasons for some of our medical issues today.